Introduction
Hydrocarbons have been produced in North Dakota for many years. The oldest natural gas, or methane, production was non-commercial production reported from a Dakota Sandstone water-well near Edgeley in 1892
(Anderson and Eastwood, 1968). Later, shallow gas was discovered in Bottineau County in sufficient quantity to supply the towns of Mohall, Westhope, Lansford, and
some small towns and farmsteads during the early 1900's but, by 1920, the produced volume had decreased so much that only a few farmsteads could still be supplied. The oldest commercial hydrocarbon production in North Dakota
was established in 1929 when the Cedar Creek gas field was extended into Bowman County from Montana. Production is from the Cretaceous Eagle and Judith River sandstones. Development of the gas continued sporadically
with wells being completed in the mid-1940's and the late 1970's to early 1980's. Less than 100 gas wells were drilled on the Cedar Creek anticline in North Dakota.
Oil is the most important hydrocarbon produced in the state, but oil was not discovered until 1951 when Amerada Hess Corporation completed the Clarence Iverson #1 on the Nesson anticline. Since then, oil exploration has
been nearly continuous in the state. The discovery well was completed in the Silurian Interlake Formation but subsequent development on the anticline focused on the Mississippian Madison Group. More than 13,000 wells have been
drilled in the state since 1951 (Fig. 1) and more than 1,300,000,000 barrels of oil have been produced (Oil & Gas Division, 1998). Annual oil production peaked in 1984 at more than 52,000,000 barrels of oil (Fig. 2) but decline
followed. The decline was temporarily reversed in the late 1990's, so much so that daily oil production briefly rose above 100,000 barrels of oil per day for the first time since 1991 in late 1997 (Fig. 3).
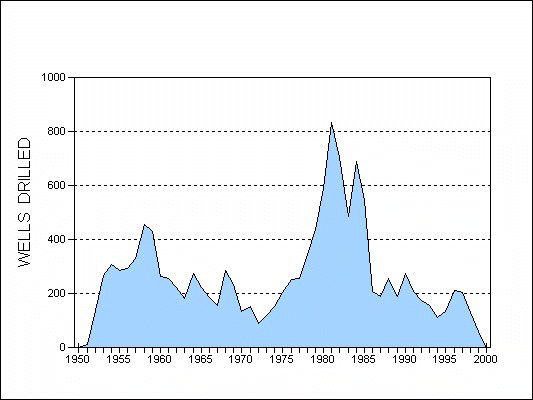
Figure 1. Number of wells drilled in North Dakota since 1951.
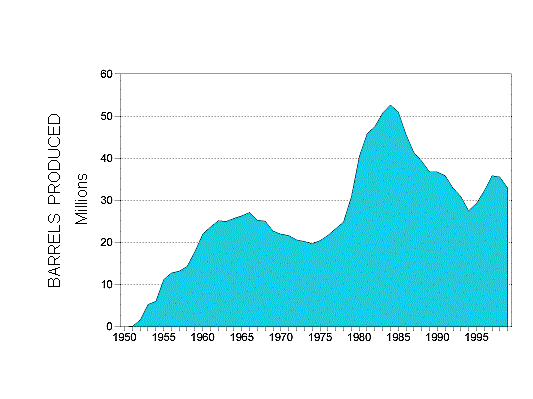
Figure 2. Total annual oil production in North Dakota.
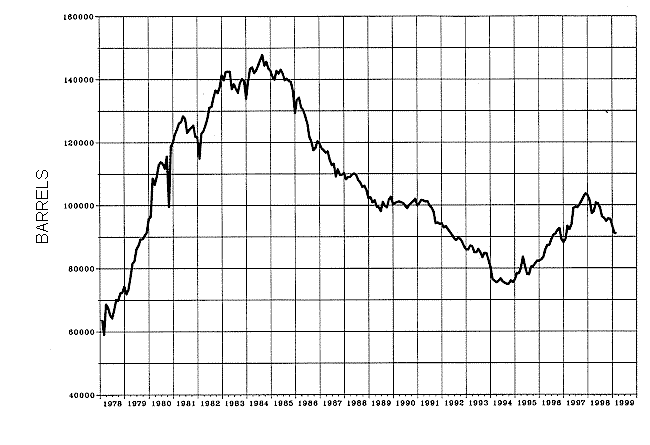
Figure 3. Average daily oil production in North Dakota (ND Oil & Gas Division).
Nationally, North Dakota tied Kansas as the 7th largest oil-producing state in America, but compared to the leading states, it is a relatively minor oil-producing state. Texas produced 1,512,000 barrels of oil
while North Dakota produced 95,000 barrels, one-sixteenth the amount, during the same period (Oil & Gas Journal, 1999).
Crude oil is a naturally occurring complex liquid hydrocarbon whose main chemical components are hydrogen and carbon. Crude oils contain many other elements and compounds, like sulphur, nitrogen, iron, or sodium
chloride (Levorson, 1967). North Dakota crude oils are generally paraffinic base (Folsom, 1973), that is they belong to the paraffinic or methane series. Most of the crude oils produced in the state range from 19 to
55 degrees of API gravity. The Third Edition of the Glossary of Geology defines a paraffin series as a homologous series of saturated aliphatic hydrocarbons, empirical formula CnH2n+2, of which methane is the lowest
and representative member, followed by ethane, propane, the butanes, etc. Some paraffinic crude oils, like in those produced from the Pennsylvanian Tyler Formation, contain so much paraffin that a solid, waxy material will
precipitate from the crude as its temperature decreases. This paraffin can be a valuable component of the crude and is most commonly found in stores as petroleum jelly. Crude oil is measured in standard barrels and one barrel
of oil contains 42 U. S. gallons.
Most of the crude oils in North Dakota also contain some natural gas. At reservoir temperatures and pressures, the gas is dissolved in the oil but, when the oil is brought to the surface, the oil and gas separate into
liquid and gas phases. When all the gas in the reservoir is dissolved in the oil at reservoir conditions it is called associated gas, and the oil is undersaturated with natural gas. If there is more natural gas than the
crude oil can contain, then the oil is saturated. In this situation, two phases of hydrocarbons can form in the reservoir, a gas phase, or gas cap, which forms in the structurally highest part of the reservoir, and a liquid
oil phase that is found underneath it. Both saturated and undersaturated reservoirs are usually considered to be oil reservoirs. If only gas is present in the reservoir then it is a gas reservoir. Most reservoirs in North
Dakota are undersaturated oil reservoirs. Gas reservoirs are rare in North Dakota as are reservoirs with a free gas cap.
Most of the natural gas found in North Dakota is "wet gas", that is it contains hydrocarbons that are liquid under pressure but a gas at surface pressures. Some of these wet gases are propane and butane and they are a
valuable by-product to the oil producers as they are usually sold separately from the crude oil and methane. Natural gas is sold in standard cubic feet measured at 60° F and 14.73 psi.
Once a well is on production, the oil and natural gas are separated at the well site. The oil is transported by either a truck or by pipeline to a refinery. The refinery at Mandan is the only operating refinery in
North Dakota and it has a daily capacity of around 60,000 barrels of crude per day. The remaining crude oil is transported out of the state to other refineries by pipeline.
Produced natural gas is either used at location, flared at location, or pipelined to a gas processing plant. Natural gas is often used to operate equipment, like pumps or treaters, at the well site. It can be a
significant savings over running power lines and buying the electricity to run the equipment. If, after lease use, the volume of gas produced is too small to justify the expense of building a pipeline to the well
site, the gas is flared, or burnt, at location. Pipelines are expensive to build, costing tens of thousands of dollars per mile to build. To justify the expense, estimates of the amount of gas contained in a reservoir
must be weighed against the distance, and thus cost, to the nearest point where a hook-up to the natural gas gathering system can be made. Oil companies drill wells to make money and if the economics aren't right, no
pipeline will be built. When enough gas is produced to justify the cost, a pipeline is built to the well and the gas is sold. The gas goes from the well to one of the nine gas processing plants in North Dakota where
gases like butane and propane are separated from the methane. The methane is then put into the regional gas pipeline system for sale and the various other wet gases are sold.
Hydrocarbon production is limited to seventeen counties in western North Dakota (Fig. 4). Some possible reasons for the limited distribution of oil and gas are: absent or immature source rocks, potential reservoir
rocks have either been eroded off or weren't deposited, the migration paths of the hydrocarbons did not cross any other counties, or hydrocarbons are present but have not yet been discovered.
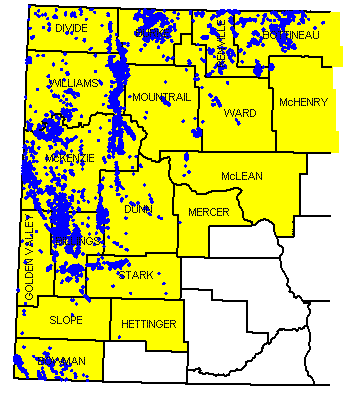
Figure 4. North Dakota oil producing counties (yellow) with the producing wells (blue).
General Geologic Setting
The Williston Basin is a large, roughly circular depression on the North American Craton covering several hundred thousand square miles across parts of North and South Dakota, Montana, and the Canadian
provinces of Manitoba and Saskatchewan (Fig. 5). The Williston Basin began to subside during the Ordovician Period (Fig. 6), around 495 million years ago and it underwent episodic subsidence throughout the rest of
the Phanerozoic Eon. The Phanerozoic Eon, that part of geologic time during which life was abundant, extends from approximately 600 million years ago to the present. Although the Williston Basin was subsiding, marine
sediments were not deposited in it continuously. The basin contains an unusually complete rock record compared to many basins, but even in the Williston Basin erosion dominated a significant amount of the Phanerozoic Era
(Fig. 7). However, some rocks from each Phanerozoic period are preserved in the basin. These strata record several cycles of marine transgressions that filled the basin, followed by marine regressions that drained the basin.
Sloss (1963) defined six inter-regional rock-stratigraphic units that were traceable across the North American craton and named them sequences. One of the example areas Sloss used when he first proposed the sequence concept
was the Williston Basin. He divided the Phanerozoic Era into six sequences which, in ascending order, are the Sauk, Tippecanoe, Kaskaskia, Absaroka, Zuni, and Tejas (Fig. 7).
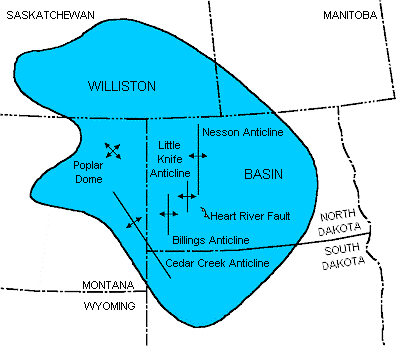
Figure 5. Extent of the Williston Basin with major North Dakota structures shown.
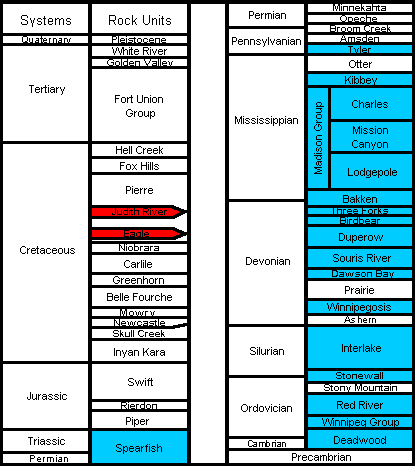
Figure 6. Generalized stratigraphic column for the Williston Basin with gas producing horizons shown in red and oil producing horizons shown in blue.
More recently, Sloss' sequence concept changed to a depositional sequence concept that is based upon the identification of sequences on seismic data (Mitchum, Vail, and Thompson, 1977).
The study of depositional sequences on seismic data has become known as seismic stratigraphy and is used worldwide as a major tool in exploration for oil and natural gas. Vail, Mitchum, and Thompson
(1977a; 1977b) also recognized that depositional sequences record regional relative sea level changes which, if coincident with relative changes in many regions, are probably of global extent. They noted three
orders of cycles and identified a number of cycles. They identified two first-order cycles, also called super cycles, that were 200-300 million years long and fourteen second-order cycles that lasted for 10 to 80
million years (Fig. 7). They also identified more than 80 third-order cycles, each lasting from 1 to 10 million years, but did not consider the pre-Jurassic cycles to be of global extent as the data came largely from
North America.
Most of the hydrocarbons are produced from rocks deposited during the Paleozoic Era (Fig. 6) although some Mesozoic strata are productive. Since the initial discovery, the Madison Group (Mississippian) has produced
the most oil, but significant volumes have also been found in the Red River (Ordovician) and Duperow (Devonian) formations (Table 1). Sandstones of the Spearfish Formation (Triassic) produce oil in north-central
North Dakota and Eagle and Judith River Sandstones (Cretaceous) produce natural gas in southwestern North Dakota. Natural gas had been produced from other Cretaceous sands in Dickey and LaMoure counties and from
glacial drift in Bottineau County, but none is produced from these strata now.
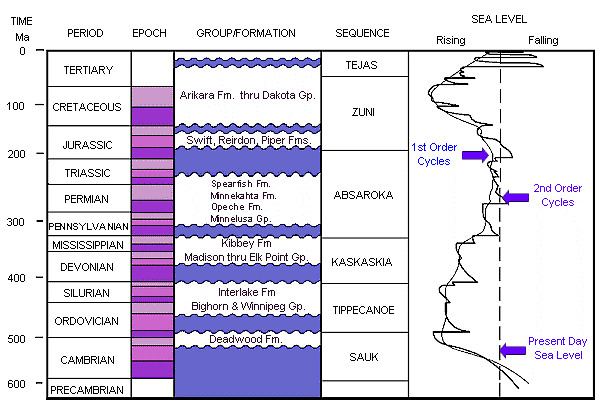
Figure 7. Time-stratigraphic column of the North Dakota Williston Basin with the First and Second Order sea level curves of Vail, et al. (Modified from Fowler and Nisbet, 1985).
Table 1- Total Production in North Dakota by Formation in stratigraphic succession (Oil & Gas Division, 1997)
Formation |
Barrels of Oil |
Ranking |
Spearfish |
421,016 |
5* |
Spearfish/Madison |
47,264,282 |
5 |
Tyler |
73,473,133 |
4 |
Madison |
765,936,439 |
1 |
Lodgepole |
12,704,599 |
7 |
Bakken (Sanish) |
40,309,285 |
6 |
Birdbear (Nisku) |
5,265,538 |
10 |
Duperow |
122,890,435 |
2 |
Souris River |
16,512 |
14 |
Dawson Bay |
3,525,218 |
11 |
Winnipegosis |
6,186,977 |
9 |
Silurian (Interlake) |
55,106,040 |
5 |
Stonewall |
9,244,665 |
8 |
Gunton |
164,146 |
13 |
Red River |
106,282,242 |
3 |
Red River "B" |
11,378,199 |
3* |
Winnipeg/Deadwood |
347,606 |
12 |
Total |
1,260,516,332 |
|
Note: Red River and Red River "B" should be added together for the Red River production total.
Structural Geology
The Williston Basin, compared to many basins worldwide, is a structurally simple basin. It is roughly circular, deepest in its center, and the strata become both shallower and thinner towards its margins.
It is a large basin, covering approximately 150,000 square miles over parts of North Dakota, South Dakota, and Montana and parts of the adjacent Canadian provinces of Saskatchewan and Manitoba. The basins
deepest point is thought to be near Williston, ND where the Precambrian surface is more than 16,000 feet below the surface.
The early structural history of the basin, from the deposition and/or emplacement of the oldest rocks, is poorly understood. The earliest rocks are difficult to study because the Lower Phanerozoic and
Precambrian rocks are not exposed at the surface in North Dakota and only a few wells have penetrated these rocks. Our present understanding of the early geologic history of the basin is pieced together from outcrops in
adjacent states and provinces, from seismic data, and from the limited well data.
Precambrian rocks under North Dakota can be placed into one of three geologic provinces, the Superior Craton, the Wyoming Craton, or the Trans-Hudson orogen (Fig. 8). The Superior Craton underlies the eastern half of
North Dakota and is a part of the North American Canadian Shield. The Superior Craton is Archean in age and consists mainly of granites and greenstones that were emplaced between 2.75-2.6 billion years ago. Underlying eastern
Montana, and possibly extending into western North Dakota, is the Wyoming Craton (Archean). Rocks of the Wyoming Province are gneissic, or banded to lenticular feldspar and quartz-rich rocks, and are approximately
the same age as rocks from the Superior Craton. Between these two cratons, under most of western North Dakota, lies the Trans-Hudson orogen, which is composed of oceanic material from an early rifting event
within the Superior Craton. A later collision between the Superior craton and the Wyoming and/or Churchill cratons accreted volcanic island-arc material to the orogen. (Green et al, 1985). Rocks from the
island-arc complexes are between 1.95 and 1.85 billion years old, or Early Proterozoic in age, while the oceanic material apparently is older, but still Proterozoic in age.
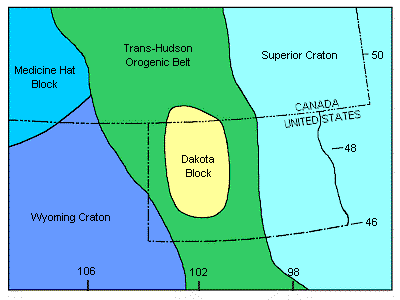
Figure 8. Structural elements in the vicinity of the Williston Basin (Modified from Green et al, 1985).
The internal structural geology of the two provinces and the orogen can be very complex, as are the structural relationships between them. Our limited ability to either sample the rocks or to seismically
image them restricts our ability to determine how the Williston Basin formed. The early structural history of the basin has had an impact on later Phanerozoic deposition, but the extent of that impact is not
always known. Although subsurface data is limited, in Beaver Lodge Field on the Nesson anticline, it can be demonstrated from well control that within a few miles, there are hundreds of feet of topographic
relief on the Precambrian surface (Fig. 9). The relief on the basement surface is either an erosional remnant of a granite knob or is a horst block formed by movement along vertical to near-vertical faults.
Most of the structural deformation during the Phanerozoic Eon in North Dakota probably resulted from the subsidence of the Williston Basin. The main evidences of structural deformation in the basin
are folding and faulting. The best evidence of folding in North Dakota is the anticlinal and synclinal structures that were formed. Some of these structures, like the Nesson, Cedar Creek, Little Knife,
and Billings anticlines, produce oil (Fig. 5). Many other unnamed anticlinal structures exist in the basin, but only some of them have been proven to be oil-producing.
The other primary evidence of structural deformation is faulting, and faults are less well documented in North Dakota. Some faults, like those on the west flank of the Cedar Creek anticline (Clement, 1987) and the Heart River
fault (Chimney et al., 1992) are visible on seismic. Clement (1987) shows faults to be steeply dipping, almost vertical, and reports that faults along the Cedar Creek anticline have undergone recurrent near-vertical and wrench
movements. He also reports that the displacement direction along some of these faults changed over time. Faults have been inferred elsewhere, but well control is insufficient to prove their existence and/or the fault's
displacement is too small to be imaged on seismic data. The inferred faults probably have similar characteristics to the larger faults on the Cedar Creek anticline, that is they dip nearly vertically, may have a component
of wrenching, have undergone recurrent movements, and the direction and magnitude of displacement has changed over time. However, it is unlikely that most faults will be proven to exist because the near-vertical dip makes
them difficult to intersect or image.
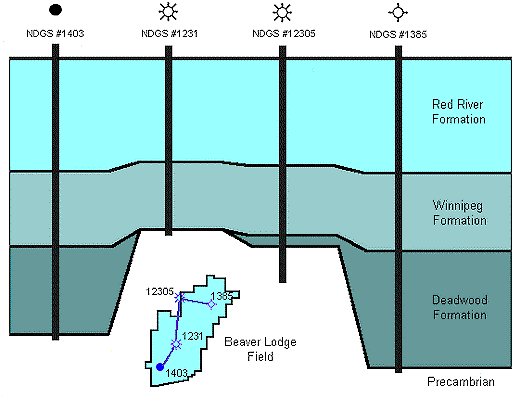
Figure 9. Cross section across Beaver Lodge Field of the Ordovician to Precambrian section. Note the relief on the Precambrian surface and the missing Deadwood section over the Precambrian high.
Features that are frequently interpreted to be present in the Williston Basin are lineaments. A lineament is the surface expression of a basement block boundary or fault (Brown and Brown, 1987).
Tectonic stresses in the Williston Basin have changed over time and these stresses may have been relieved through vertical and/or lateral movement along pre-existing zones of weakness like a fault.
The magnitude of individual displacements may not have been great, but the displacement was at least sufficient to have affected local deposition and erosion patterns. These local patterns often control where
oil is trapped.
Stratigraphy and Petroleum Characteristics
Basement Rocks (Precambrian)
Basement is the crust of the earth extending from the base of sedimentary cover down to the Mohorovicic discontinuity, or all Precambrian rocks. Phanerozoic deposition in the Williston Basin began on a
surface of weathered basement rocks.
The Precambrian is subdivided into two eons. The older is the Archeozoic Eon, from between 4 to 2.5 billion years while the younger, Proterozoic Eon occurred between 2.5 to 0.670 billion years. The
geology of the Precambrian rocks underlying the Williston Basin is complex, consisting of many juxtaposed, fault-bounded lithostructural domains (Peterman and Goldich, 1982). Green et al (1985) suggested
that the basement rocks in western North Dakota formed in an orogenic belt, or linear deformed area, called the Trans-Hudson orogen that lies between the Archean Superior and Wyoming Provinces (Fig. 8). More
recently, Baird and others (1996) reported another, previously unknown block under western North Dakota which they named the Dakota block. They interpreted the block to be an Archean continental fragment
because of similarities in its reflection geometry to Archean crustal material exposed in the Glennie domain in Canada. In general, basement rocks do not produce oil in North Dakota, but one well in
Newporte Field did produce oil from fractured Precambrian rocks.
Sauk Sequence (Cambrian-Lower Ordovician)
The Upper Cambrian Deadwood Formation records both the earliest Phanerozoic sedimentation and the beginning of the Sauk Sequence in the Williston Basin (Fig. 6). The Cambrian sea transgressed eastward into an
embayment on the edge of the Cordilleran shelf (Carlson, 1960; Lochman-Balk, 1972), and deposited siliciclastic sediments, sands and shales, as the dominant sediment type in North Dakota. During Lower
Ordovician, carbonate sediments began to be deposited in the center of the basin, which was now formed and had begun to subside (LeFever et al., 1987). The Cambrian Deadwood Formation produces oil along
the Nesson anticline, in eastern Williams and McKenzie counties, and in Newporte Field in Renville County (Fig. 10).
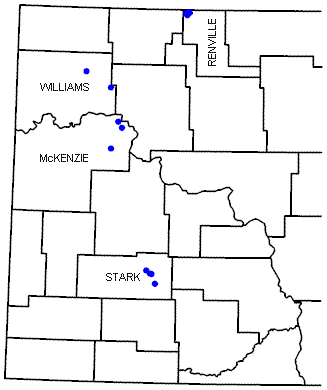
Figure 10. Location of oil and gas wells producing from the Cambrian Deadwood and Ordovician Winnipeg formations.
Tippecanoe Sequence (Ordovician-Silurian)
The Williston Basin contains a well-preserved Ordovician section for the Rocky Mountain region (Foster, 1972). Equivalent strata are thought to have covered a much greater area, once extending at
least as far as Nebraska to the south and southeast, but later erosion has removed much of the strata. Deposition was continuous across the Ordovician-Silurian boundary and sedimentation continued at
least until Middle Silurian. The top of the sequence is a major erosional unconformity that has removed an unknown amount of strata.
Winnipeg Group
Earliest Tippecanoe Sequence (Fig. 7) sedimentation begins with the Williston Basin connected to the ocean through a southwest trending seaway (Foster, 1972). Sedimentation is recorded by the
Black Island, Icebox, and Roughlock formations of the Winnipeg Group. All of these formations were deposited in marginal to shallow marine environments.
The Black Island Formation has two members (Thompson, 1984). The lower member is comprised of two lithofacies, a lower red-bed lithofacies containing quartz arenites and "clayshales", and an upper
green quartz wacke. The upper member is also comprised of two lithofacies, a quartz arenite and green quartz wackes. The Icebox Formation, an organic-rich green shale, is thought to be a source rock
for Lower Paleozoic reservoirs (Dow, 1974; Williams, 1974). The Roughlock Formation is predominantly a nodular limestone and is transitional with the overlying Red River Formation (LeFever et al, 1987).
The Winnipeg Group is productive on the Nesson anticline and at Richardton and Taylor fields, on the Heart River anticline in eastern Stark County (Figs. 5 & 9). In both areas, production is from
Black Island sandstones and natural gas is the dominant hydrocarbon produced.
Bighorn Group
The Big Horn Group conformably overlies the Roughlock Formation of the Winnipeg Group (Fig. 6). The Red River Formation, the basal unit of the group, has been subdivided into two informal members.
The lower member is the lower two-thirds of the formation and is composed of fossiliferous and selectively dolomitized limestones. The upper member includes four dolomitized porosity zones; the "D",
"C", "B", and "A" zones, in ascending order (Carroll, 1979). The upper three zones are capped by anhydrite beds while the "D" zone is not (Fig. 11). The anhydrites are widespread, as they are present
across most of western North Dakota and eastern Montana, and they are present in the center of the basin (Fig. 12). Two depositional models have been proposed to explain the origin of the dolomites and
capping anhydritesin the upper Red River Formation. In the first model, the marine waters in the central Williston Basin evaporated to the point that a sabkha developed there (Carroll, 1979) while in the
second model, all deposition occurred in subtidal environments (Kendall, 1976). The data can be interpreted to fit either model, hence the controversy.
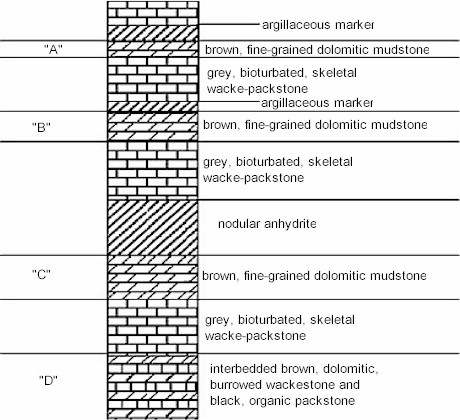
Figure 11. Stratigraphy of the upper Red River Formation The letters correspond to informally named porosity zones. (Modified from Carroll, 1979).
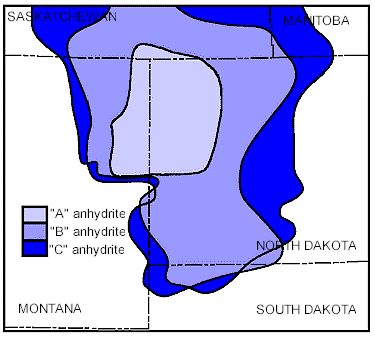
Figure 12. Distribution of the Red River "A", "B", and "C" anhydrites (Modified from Foster, 1972).
In the sabkha model, the "D" zone strata were deposited in a subtidal to intertidal environment and the basal strata of the "C", "B", and "A" zones were deposited in a subtidal environment.
The upper strata of the "C", "B", and "A" zones were deposited in a broad supratidal environment, or sabkha (Carroll, 1979). In this model, a series of marine transgressions deposited subtidal to
intertidal sediments during the basal phase of each cycle, were followed by a marine regression. In the cases of the "C", "B", and "A" zones, the regression dried out the basin. During "D" zone sedimentation,
fresh water infiltrated into the sediments on the tidal flats and mixed with normal marine waters changing the Mg/Ca ratio of the mixed waters enough to dolomitize the sediments. Dolomitization in the upper
three zones was different - penecontemporaneous with deposition. The anhydrites were deposited in the high-evaporation environment of the sabkha.
The sabkha model requires that sabkha progradation continued until the center of the basin was completely filled. In the second model of upper Red River sedimentation, deposition occurred in a subtidal
environment. In this model, the dolomites and anhydrites were deposited during periods of basin restriction and increasing water salinity (Kendall, 1976). The anhydrites were precipitated from the seawater
when a high enough water salinity was reached before conditions returned to a more normal marine environment. Dolomitization would occur because the Mg/Ca ratio of the water increased with the precipitation of
the anhydrite and then dolomitization could occur. Another possibility is that the dolomites formed as a result of a later diagenetic event.
The Red River Formation is productive across most of western North Dakota. Most Red River Formation production has been found west of the Nesson anticline, in the deepest parts of the basin, and is associated
with structural closures. However, the best porosity is not always coincident with the structure's crest (Longman et al, 1983). The Red River Formation is the second most important hydrocarbon producing horizon in
North Dakota and produces hydrocarbons in many fields (Fig. 13). The Stony Mountain Formation conformably overlies the Red River Formation and is comprised of interbedded calcareous shales and argillaceous
limestones. The Stony Mountain Formation is rarely productive, but where it is productive it is always associated with a Red River Formation structure. Production usually comes from the Gunton Member which can
have a well developed dolomite porosity. The Stonewall Formation is the uppermost formation in the Big Horn Group and conformably overlies the Stony Mountain Formation (Fig. 6). Continuous sedimentation occurred across
the Ordovician-Silurian boundary and dolomites and limestones, with thin anhydrite beds near the basin center, were deposited. The Stonewall Formation produces oil and gas from several zones, usually associated with a Red
River structure (Fig. 14).
Interlake Formation
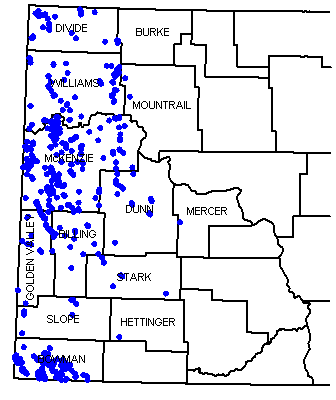
Figure 13. Partial distribution of oil and gas wells producing from the Ordovician Red River Formation.
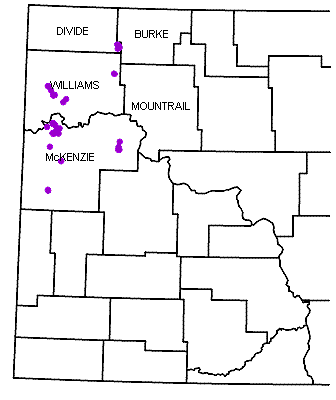
Figure 14. Partial distribution of oil and gas wells producing from the Silurian Stonewall Formation.
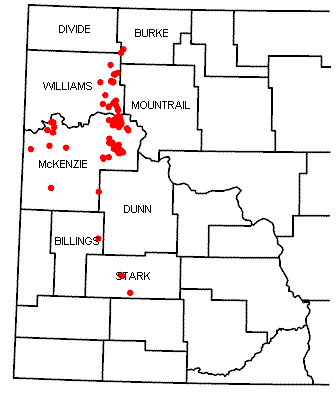
Figure 15. Partial distribution of oil and gas wells producing from the Silurian Interlake Formation.
The Interlake Formation conformably overlies the Stonewall Formation (Fig. 6) and records latest Tippecanoe Sequence deposition. Interlake lithologies are dominated by dolomitic mudstones and dolomites.
The formation was exposed from Late Silurian through Early Devonian when karst topography was formed. Various interpretations have been made of Interlake stratigraphy. LoBue (1983) informally subdivided the Interlake
Formation into three members, and interpreted the formation as a sequence of sublittoral to supralittoral environments. LoBue also recognized paleosols, and interpreted them as periods of prolonged subaerial exposure.
Megathan (Megathan 1987) assigned group status to the Interlake and defined eight formations within it. Megathan interpreted Interlake Group deposition as occurring in a succession of hypersaline (lower Interlake)
to freshwater (upper Interlake) environments. In contrast, Inden et al (Inden et al 1988) considered the Interlake to be a formation and interpreted it as a series of low-energy, shallowing-upward cycles deposited in
a restricted-marine environment.
The upper Interlake Formation is productive along large structures (Fig. 15), but the controls on production are not well understood. Salt-plugged porosity degrades reservoir performance in some places whereas
fracturing has enhanced performance in other areas. The middle Interlake Formation is productive in two fields in Stark County and the lower Interlake Formation produces from two porosity zones, informally named
the Salsbury and the Putnam. These two porosity zones produce on major structures in North Dakota and production can be significant, like that at Stoneview Field on the Nesson anticline. Typically, oil with a
significant volume of gas is produced from Interlake reservoirs.
Kaskaskia Sequence (Devonian-Mississippian)
Kaskaskia Sequence deposition in the Williston Basin occurred during two transgressive cycles, so the sequence is divided into two parts. Limestones dominate the Kaskaskia Sequence rock-record, but two
major evaporate sections are preserved. Rocks of the lower cycle record a northwest connection into the Elk Point Basin while deposition in the upper cycle records a westward connection into the
Central Montana Trough.
Lower Kaskaskia Sequence
Elk Point Group
The initial Kaskaskia Sequence transgression into the basin was from the northwest out of the Elk Point Basin (Fig. 16). At the base of the sequence is the Ashern Formation. Lobdell (1984) divided the
formation into two members, a lower red dolostone and an upper gray dolostone. The lower member was deposited in a restricted marine environment, whereas the upper member records a change to a less restricted
environment as marine transgression continued. Both nodular and bedded anhydrite are present throughout the Ashern, but are more common in the lower member. The Ashern Formation is non-productive in North Dakota.
The Winnipegosis Formation conformably overlies the Ashern Formation (Fig. 6) and is dominantly a limestone. In northwestern North Dakota, initial Winnipegosis deposition occurred in the southeastern extent of the
Elk Point Basin and on a broad shelf ramp (Fig. 16).
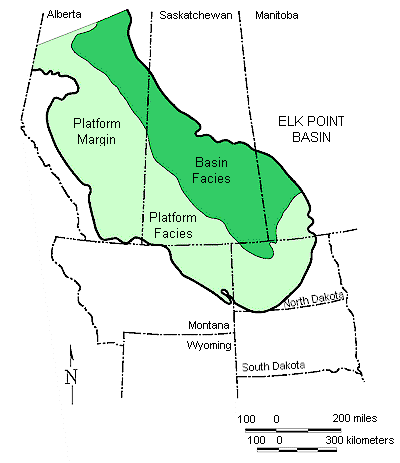
Figure 16. Extent of the Winnipegosis platform and basin in the Elk Point Basin (Modified from Fuzesy, 1982 and Ehrets and Kissling, 1987).
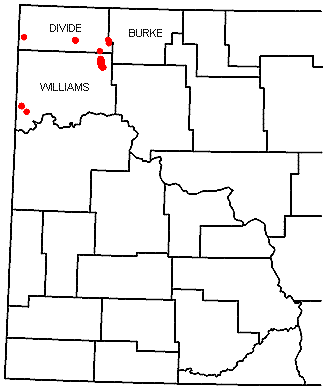
Figure 17. Partial distribution of oil and gas wells producing from the Devonian Winnipegosis Formation.
Later, the shelf differentiated into a basin surrounded by a platform (Ehrets and Kissling, 1987). Shallow water mounds grew on the shelf but, when the shelf differentiated into a basin and platform,
the mound biota struggled to keep up with increasing water depths in the rapidly subsiding basin. Eventually, some of the mounds grew into pinnacle reefs.
Some pinnacle reefs produce commercial quantities of oil in the Canadian portion of the Williston Basin, but none do in North Dakota. Commercial Winnipegosis production has been established in North Dakota
along the platform margin, at Temple and Hamlet fields, and on the platform, in Round Prairie Field (Fig. 17). Platform margin fields produce from argillaceous carbonates deposited in basin-slope facies;
platform fields produce from patch reefs (Ehrets and Kissling, 1987). The Winnipegosis Formation produces oil with a large volume of natural gas.
During latest Winnipegosis deposition, the basin became restricted and the evaporites of the Prairie Formation were deposited. With time, salt deposition spread from the basin onto the basin margins.
The restriction continued until eventually, the pinnacle reefs became encased in salt (Kerr, 1988). Dissolution of the Prairie salt is an important local trapping mechanism in the Williston Basin. Beds
draped across dissolution edges enhanced closure, as exhibited by Glenburn, Sherwood, and Wiley fields, while two-stage salt dissolution formed the "Nisku Reefs" of northeastern Montana.
Manitoba Group
When the northern seaway into North Dakota reopened and normal marine circulation was reestablished, the Dawson Bay Formation was deposited on a stable, low-relief shelf. In northwestern North Dakota,
stromatoporoid-dominated patch reefs formed on an open platform (Dean, 1982). Anhydrite beds in the upper Dawson Bay Formation record renewed restriction of the seaway into the Williston Basin.
The Dawson Bay Formation has produced oil in three North Dakota fields (Fig. 18). At Dolphin and Temple fields, both significant oil fields, porous carbonates pinch out updip on a structural nose
(Dean, 1982; Heck, 1987). At Marmon Field, the trap is essentially the same as at Dolphin and Temple fields, but the volume of oil produced is insignificant. In western North Dakota, porosity in the
Dawson Bay Formation is generally salt-plugged. However, the localized dissolution of the pore-filling salt can form or enhance a stratigraphic trap as is the case in Dolphin Field. Dawson Bay production
is primarily oil, but with a significant volume of associated gas. The Souris River Formation (Fig. 6) conformably overlies the Dawson Bay Formation, and is lithologically similar to it. The formation's
interbedded carbonates and evaporites are evidence that the marine restriction, begun during latest Dawson Bay deposition, continued into Souris River deposition.
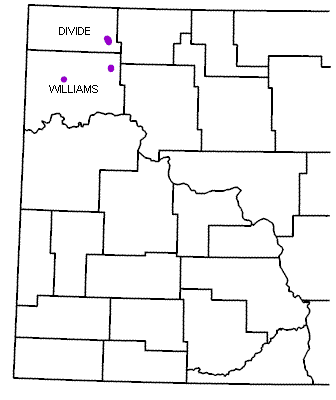
Figure 18. Partial distribution of oil and gas wells producing from the Devonian Dawson Bay Formation.
The Souris River Formation is not considered to be an important oil producing zone because oil has been produced from only one well in Dolphin Field. However, an unknown amount of Souris River Formation
production along the Nesson anticline was pooled with that from the overlying Duperow Formation (Pilatzke et al., 1987).
Jefferson Group
The Duperow Formation conformably overlies the Souris River Formation (Fig. 6). Two hypotheses have been proposed to explain the changes in lithology from a lower limestone to dolomite to an upper anhydrite
observed in the Duperow Formation. The first model is a series of shallowing-upward sequences where the depositional environment changed from a lower subtidal environment to a middle, restricted environment
and ultimately, to an upper intertidal to supratidal environment (Wilson, 1967; Pilatzke et al., 1987). Lithologies similarly changed from burrowed bioclastic limestones containing stromatoporoid banks, to
lime mudstones containing ostracods and calcispheres, to bedded anhydrites and silty dolomites.The second model invokes a series of marine restrictions during which salinity increased. Each restriction began
in normal to near normal marine environments and culminated in a hypersaline environment in which bedded anhydrites and dolomites were deposited (Ehrets and Kissling, 1985). This model has sometimes been called
a brining-upwards sequence.
The Duperow Formation produces from stratigraphic traps in the central Williston Basin, from structural traps along the Nesson anticline, and from combination traps on the Billings anticline. The Duperow Formation
also produces on the eastern flank of the Cedar Creek anticline, where truncated porous carbonates are capped by Englewood Formation equivalents. The Duperow Formation is the third largest oil producing zone in the
state, after the Madison Group and the Red River Formation, and produces a combination of oil and natural gas (Fig. 19).
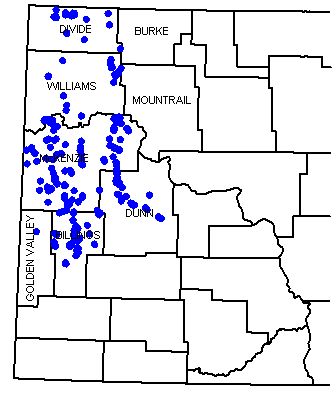
Figure 19. Partial distribution of oil and gas wells producing from the Devonian Duperow Formation.
The Birdbear (Nisku) Formation conformably overlies the Duperow Formation. Loeffler (1982) described the Birdbear Formation as fossiliferous limestones and dolomitic muddy limestones deposited in shallow, normal
marine environments, that changed upwards into anhydrites and dolomites deposited in a sabkha environment.
The Birdbear Formation produces oil and natural gas from a variety of traps. Stratigraphic traps include stromatoporoid banks, amphiporid back-bank facies, and locally dolomitized porosities. The formation also
produces from small structures on the Nesson anticline and elsewhere (Fig. 20), truncated porosity zones along the east flank of the Cedar Creek anticline, and from two-stage salt dissolution structures in
northeastern Montana.
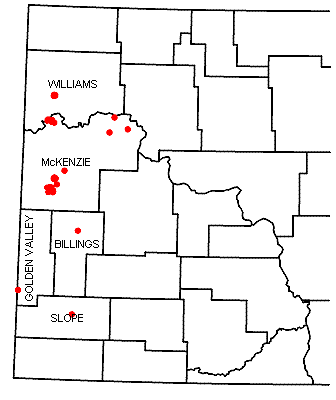
Figure 20. Partial distribution of oil and gas wells producing from the Devonian Birdbear Formation.
Three Forks Formation
The Three Forks Formation conformably overlies the Birdbear Formation (Fig. 6) and was deposited in shallow marine to supratidal environments in a shallow epeiric sea (Dumonceaux, 1984).
The Three Forks Formation is primarily a micrite to dolomicrite interbedded with anhydrite. An informal unit, called the Sanish sandstone, can be developed at the top of the Three Forks Formation.
The Sanish sandstone produces oil and gas in Antelope Field and is one of the largest oil pools in the state.
Bakken Formation
The Bakken Formation conformably overlies the Three Forks Formation in the basin center, and unconformably overlies it elsewhere (Webster, 1984). Gerhard et al. (Gerhard et al. 1982) thought the
Bakken Formation recorded the initial depositional phase of the upper Kaskaskia Sequence. However, Hester and Schmoker (Hester and Schmoker 1985) showed that the Bakken Formation depositional pattern
was coincident with the Elk Point Basin. Therefore, the Bakken Formation is included in the lower Kaskaskia Sequence.
The Bakken Formation has three informal members, an upper and a lower black, organic-rich shale, separated by an arenaceous limestone to siltstone. Depositional environments interpreted for the Bakken
Formation have ranged from a marine swamp with restricted circulation caused by the prolific growth of organic matter to an offshore marine environment with a stratified water column (LeFever, 1991). The
latter environment model is preferred.
The Bakken Formation is an excellent source rock and was considered to be the source rock for most Mississippian reservoirs in the Williston Basin (Dow, 1974; Webster, 1984; Price et al., 1984).
Recent work has shown that the Lodgepole Formation was the source of at least some of the Mississippian oils (Osadetz and Snowdon, 1986). Price and LeFever (1994) determined that the Bakken oils were
confined to the Bakken source system (uppermost Three Forks Formation to lowermost Lodgepole Formation), and have not migrated into the overlying Mississippian reservoirs as previously thought.
The Bakken Formation is productive on, and near, the Nesson anticline, and along its southwestern depositional limit in Golden Valley and Billings counties (Fig. 21). The upper black shale of the Bakken
Formation has poor matrix permeabilities but produces where overpressured and fractured. Production from the middle member is found along the northern Nesson anticline where low porosity and permeability
sandstones produce some oil.
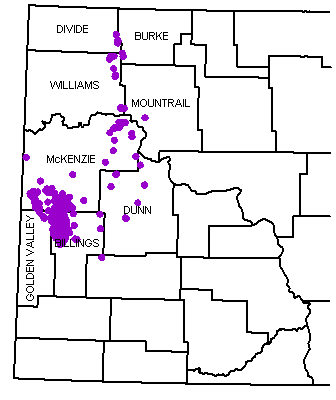
Figure 21. Partial distribution of oil and gas wells producing from the Mississippian-Devonian Bakken Formation.
Upper Kaskaskia Sequence
Madison Group
Deposition of the upper Kaskaskia Sequence began sometime after middle Lodgepole deposition (Fig. 22). At that time, the sedimentation in the basin records a change in sediment source from the Elk Point
Basin to the Central Montana Trough (LeFever and Anderson, 1984).
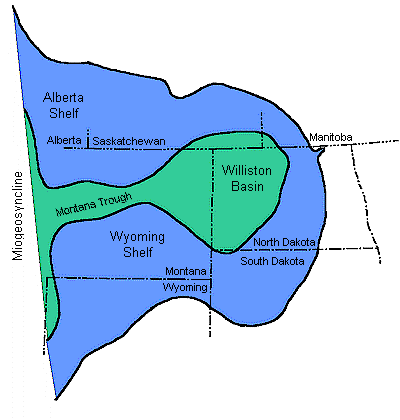
Figure 22. Seaway connection of the Williston Basin to the ocean during Upper Kaskaskia deposition (Modified after Gerhard et al., 1982).
The Madison Group is made up of three formations; the Lodgepole, Mission Canyon, and Charles, in ascending order (Fig. 6). These formations are conformable in the basin center, but exhibit complex
intertonguing relationships along the basin margins.
The Madison Group has been divided into a number of informal, wireline log-defined intervals (Harris and others, 1966; Voldseth, 1986). In ascending order, they are the Bottineau, Tilston, Landa,
Wayne, Glenburn, Mohall, Sherwood, Bluell, Coteau, Dale, Nesson, Midale, Ratcliffe, and Poplar intervals. Hendricks (Hendricks-1988)subdivided the Ratcliffe interval into several subintervals, the Berentson,
Alexander, Flat Lake, Charles C, Lustre, and Eggebrecht (Fig. 23).
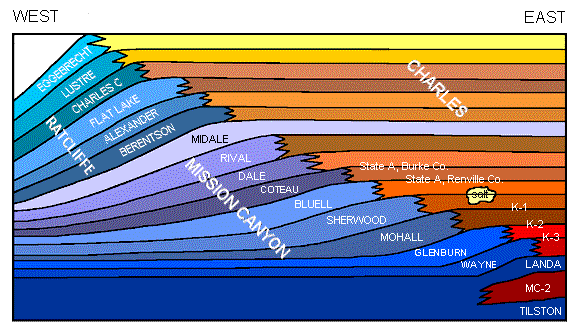
Figure 23. Facies relationships and nomenclature of the Mission Canyon and Charles formations in the Williston Basin (Modified from Hendricks, 1988; Voldseth, 1986; Harris et al., 1966).
The blue colors represent carbonate rocks and the reds and yellows represent evaporites.
The Lodgepole Formation (Bottineau interval) conformably overlies the Bakken Formation in the basin center, and unconformably onlaps Upper Devonian strata in both eastern North Dakota and along
the Cedar Creek anticline. The formation consists of limestones and dolomites deposited in normal marine to restricted shelf environments (Heck, 1979).
The Lodgepole Formation is a major producing horizon in Manitoba, but significant production in North Dakota was not found until 1994 when the Dickinson Lodgepole pool was discovered. Lodgepole wells
in this pool, and several other nearby fields, are capable of producing several thousand barrels per day from Waulsortian bioherms. Bjorlie and Anderson (1978) had postulated a system of lower Lodgepole
Waulsortian bioherms in eastern North Dakota deposited in a shallow, normal marine shelf environment, but the existence of Waulsortian bioherms in a basinal environment was completely unknown before the
Dickinson Lodgepole discovery. A porosity zone in the middle Lodgepole has produced oil in several Williams County wells, but it was uneconomic (LeFever and Anderson, 1984). In addition to containing good
reservoir rock in the Waulsortian bioherms, shale beds and argillaceous limestones in the lower Lodgepole may be an important petroleum source rock (Osadetz and Snowdon, 1986).
The Mission Canyon Formation (Tilston through Dale intervals) consists primarily of limestones interbedded with anhydrites and dolomites. Deposition occurred in environments that ranged from open marine
to coastal sabkha and record a regressive sequence (Lindsay, 1988). The Mission Canyon Formation has produced more oil than any other stratigraphic unit in the Williston Basin (Table 1 and Fig. 24).
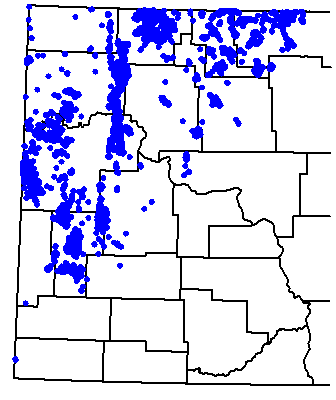
Figure 24. Partial distribution of oil and gas wells producing from the Mississippian Madison Group.
The Charles Formation (Ratcliffe and Poplar intervals) consists of interbedded evaporites and limestones deposited in a restricted marine environment.
The Charles Formation records a major marine regression during the upper Kaskaskia Sequence. Lindsay (Lindsay 1988) and Hendricks (Hendricks1988) identified four main types of
Mission Canyon traps: 1) combination structural and stratigraphic traps; 2) porous carbonate (usually an island or shoal) pinching out updip into impermeable (intertidal or inter-island) carbonate;
3) porous carbonate facies changing updip into impermeable anhydrite; 4) truncated porous carbonate capped by impermeable Triassic rocks. Approximately 60% of the oil produced in North Dakota has come
from the Charles and Mission Canyon formations.
Big Snowy Group
Latest Kaskaskia Sequence deposition is recorded by the Kibbey and Otter formations (Fig. 6). Both formations consist of interbedded sandstones, shales, and limestones. The clastic rocks had an
extra-basinal source and mark the influence of the Ancestral Rocky Mountain orogenic event (Gerhard et al., 1982). The Kibbey Formation is productive along the Weldon fault in Montana and from one
well in Red Wing Creek Field, North Dakota. In central North Dakota, the unconformity at the top of the Kaskaskia Sequence truncated only the Otter Formation. Elsewhere, variable amounts of Kaskaskia
Sequence strata are missing.
Absaroka Sequence
During Absaroka deposition, marine transgressions were from the southwest and deposition was concurrent with tectonic activity southwest of the Williston Basin. Interbedded marginal marine evaporates
and terrestrial rocks record sedimentation within the basin.
Minnelusa Group
Deposition of the Tyler Formation (Pennsylvanian) occurred in a slowly subsiding basin and recorded the beginning of the Absaroka Sequence. Sturm (Sturm 1982) divided the Tyler Formation into two
informal units, a lower unit of interbedded shales, mudstones, and sandstones; and an upper unit of interbedded limestones, calcareous mudstones, and anhydrites. He interpreted the lower unit as a
prograding delta and the upper unit as a barrier island. The latter is capped by rocks deposited in lagoonal and estuarine environments.
The Tyler Formation produces oil from both the barrier island and from channel-fill sandstones and is limited mostly to southwestern North Dakota (Fig. 25) The source rocks for this oil are thought to be
the shales within the Tyler (Williams, 1974) but Sturm (Sturm-1982), suggested that some shale and limestone beds should be examined for their source potential.
Spearfish Formation
The youngest oil-producing formation in North Dakota is the Triassic Spearfish Formation (Fig. 6), which unconformably overlies the Madison Group across much of eastern North Dakota.
The Spearfish Formation is productive where oil has migrated into it from Madison Group strata.
ZUNI AND TEJAS SEQUENCES
Dakota and Montana Groups
Zuni and Tejas Sequence strata are the youngest rocks to produce hydrocarbons in North Dakota and they produce natural gas (Fig. 6). Zuni Sequence gas production includes small volumes of gas produced from the
Newcastle Sandstone (Lower Cretaceous), in LaMoure and Dickey counties, and large volumes of gas from the Eagle and Judith River sandstones (Upper Cretaceous) of the Pierre Formation in Bowman County. These two
sandstones produce from thin interbeds of silty sandstone between shales along the Cedar Creek anticline in southwestern North Dakota (Fig. 26). This production was established during the 1920's, making it some of
the oldest production in the state (Anderson and Eastwood, 1968).The first hydrocarbons produced in North Dakota came from the youngest strata in the state, glacial drift of the Tejas Sequence. Production was
discovered in 1907, near Westhope, and other accumulations were later found near Maxbass and Mohall with enough natural gas being produced to supply the towns of Lansford and Westhope for a time (Bluemle, 1996).
However, there is no production from glacial drift today.
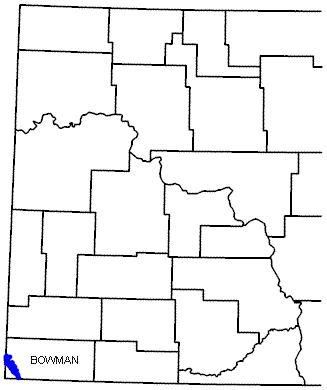
Figure 26. Partial distribution of gas wells producing from the Cretaceous Eagle and Judith River sandstones.
History of Oil and Gas Exploration in North Dakota
Oil and gas exploration in North Dakota has been cyclical with four cycles of exploratory drilling (Fig. 27). The first drilling cycle began in 1951, with the discovery of oil in Williams County.
Subsequent drilling defined the Nesson anticline, a 75-mile long structure with nearly continuous production from multiple pay zones. Two major plays, identified in 1953 and 1954, are the Mississippian
subcrop play in north-central North Dakota and the Mississippian/Pennsylvanian play in southwestern North Dakota. By the early 1960's, the first exploration cycle had ended and activity focused on
development and extension drilling.
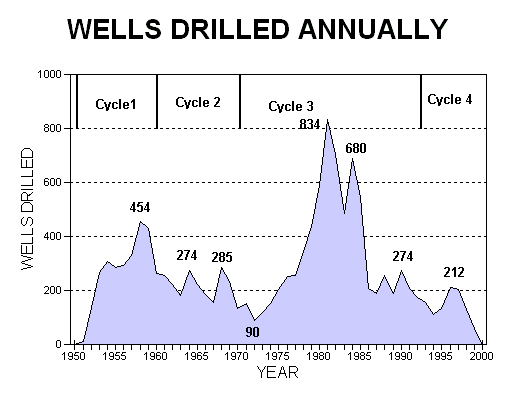
Figure 27. Wells drilled annually in North Dakota during the period 1951-1977 (four drilling cycles shown).
The second drilling cycle was in full swing by 1968 following the discovery of shallow Cretaceous oil at Bell Creek Field in the northeastern Powder River Basin. This cycle records the most exploration
for Cretaceous targets in North Dakota, and was a response by the industry to the Bell Creek Field discovery.
While the Cretaceous play in North Dakota was not successful, oil was discovered in the Red River Formation in Bowman County and from the Bakken Formation in Billings County during cycle two.
While important during cycle two, both discoveries would have a greater influence on cycle three drilling.
The third exploratory cycle began in the mid-1970's and was the most intensive of the three cycles. Hundreds of new fields and pools were discovered during this cycle with much of the drilling concentrated in
west-central North Dakota. The intensity of this cycle was the result of many factors. Two of them were the 1972 discovery of Red Wing Creek Field and the 1973 Arab oil embargo.
Red Wing Creek Field is structurally complex, with a pay section greater than 1,000 feet thick. The discovery of this field initiated a major lease play in western North Dakota. Geological and geophysical programs
were completed over many of these leases when in 1973, the oil embargo focused the industry on domestic exploration. During the cycle, no new Red Wing Creek fields were found, but the rapid escalation of oil prices
made almost any discovery economical. Some of the fields discovered during this cycle are Mondak Field, Little Knife Field, and the Billings anticline complex. These fields produce primarily from Mississippian reservoirs,
though all produce from multiple pays, and are the largest oil fields ever discovered in the state. The collapse of oil prices in 1986 brought a rapid and devastating conclusion to cycle three.
Cycle three saw the first Bakken Formation play. Previously, Bakken completions were primarily for salvage, but several Billings County completions in the early 1980's with high initial potentials focused attention on
the formation. Development of Bakken reservoirs occurred in Elkhorn Ranch, Buckhorn, and Devil's Pass fields.
Cycle four was initiated by two events, the discovery of the Dickinson Lodgepole Field and the discovery of the horizontally drilled Red River "B" pool in Cedar Hills Field in Bowman County. Cycle four was
short-lived as the Lodgepole play has not yet been successfully extended outside the immediate Dickinson area and Cedar Hills Field in Bowman County has been fully developed.
North Dakota is presently in a situation similar to that at the end of cycle three. Drilling remains flat overall, but smaller peaks are seen when a new play is active and lows are seen when there is no active play
and crude oil prices are low as they were during mid-1999.
In summary, Cycle one drilling explored mainly for reservoirs along the Nesson anticline; Cycle two activity reflected the northward extension of the Bowman County Red River play and the influence of the discovery of
Bell Creek Field; Cycle three drilling was concentrated in the central basin for deep multiple-pay targets and drilling ended when oil prices plummeted during 1986; and Cycle four was a burst of activity centered on
two plays, the Lodgepole and horizontal Red River "B" plays. Drilling will probably not increase significantly without a major price increase or a significant new discovery.
Chronology of Major Events in North Dakota
1890's |
First reported production of hydrocarbons in North Dakota.
Natural gas in artesian wells (Cretaceous) near Edgeley, North Dakota (Dickey, LaMoure, Stutsman counties).
|
1907 |
Shallow gas discovered from glacial till in Bottineau County |
1929 |
Cedar Creek Cretaceous gas play extends into Bowman County |
1951 |
Discovery of oil on the Nesson anticline |
1953 |
Definition of the stratigraphic play in Bottineau & Renville counties
Discovery of Madison oil in the Fryburg area, Billings County
|
1954 |
Discovery of Tyler oil in the Fryburg area, Billings County |
1957 |
Burke County Madison play active |
1958 |
Oil discovered on the North Dakota portion of the Cedar Creek anticline |
1961 |
Elkhorn Ranch Field Bakken pool discovered |
1967 |
Vertical Red River play in Bowman County discovered |
1972 |
Red Wing Creek Field discovered |
1976 |
Mondak Field discovered |
1977 |
Little Knife Field discovered |
1978 |
Billings "Nose" anticlinal complex discovered |
Enhanced Oil Recovery
Enhanced Oil Recovery (EOR) encompasses a variety of projects that oil companies employ to increase oil production from a field. These efforts are most often begun when an oil field is near
the end of its primary production life. Thus, other names for EOR are secondary recovery or tertiary recovery projects. A typical North Dakota oil field produces only 15-20% of all the oil trapped
in the rocks or 15-20% of the original-oil-in-place (OOIP). If conditions are right, an oil company can begin injecting water to displace the trapped oil with a waterflood, or they might drill additional
wells in an infill-drilling program to produce some of the 80-85% of the oil that otherwise would be left behind. There are other methods that can be used, but which method will be most effective will depend
on the properties of the reservoir. Some of these properties are porosity, permeability, grain size, reservoir pressure, depth, temperature, oil viscosity, and other factors. EOR projects require additional
spending by the oil companies. EOR projects can be a good investment because there are fewer risks involved as you already know the oil is there, however there is no guarantee the project will work well enough
to get the investment back.
Enhanced oil recovery (EOR) projects in North Dakota have met with varying degrees of success. Some failed to produce any incremental oil while others successfully increased recovery. Most of the unsuccessful
EOR projects were attempts to waterflood Madison reservoirs in north-central North Dakota. The failure of these waterfloods is inexplicable because waterfloods in the same strata in Canada have been successful.
One explanation is that project operations were conducted differently while another explanation is that reservoir properties in North Dakota differ from similar reservoirs in Canada. Carbonate reservoirs are often
inhomogeneous and only a thorough understanding of the reservoir characteristics and careful planning can compensate for these inhomogeneities. The EOR projects attempted in North Dakota are listed in Table II.
Each of the listed EOR projects has a unique identifying abbreviation that corresponds to those in figure 28, a location map of all the active units in North Dakota.
In 1983, Chevron Oil Co. attempted to unitize Little Knife Field to institute a CO
2 flood for pressure maintenance. A successful pilot study involving five wells had shown that the program would probably be
successful (Desch et al., 1984), but the unitization attempt failed because the 80% of the royalty interest owners necessary to ratify a unitization agreement in North Dakota was not attained.
Recent CO
2 enhanced recovery programs in Canada have apparently been successful. These successful programs, coupled with the apparent success of the Chevron pilot program at Little Knife Field,
might point to a future need for CO
2. There are two sources of CO
2 presently available to operators in the Williston Basin. The first source is the Wyoming Thrust Belt, where CO
2 is produced together with
other natural gasses. The second source is the coal gasification project at Beulah, North Dakota where CO
2 is a byproduct of the process. A pipeline is being built to transport CO
2 to the Weyburn Field in
Saskatchewan and startup of a CO
2 flood may begin during 1999. The pipeline's route takes it past many other fields that are suitable for CO
2 programs and perhaps some other fields will be
CO
2-flooded soon.
Source: North Dakota Oil & Gas Division
References Cited
Anderson, S. B., and Eastwood, W. P., 1968, Natural gas in North Dakota, in Natural Gases in rocks of Paleozoic age, American Association of Petroleum Geologists, Memoir No. 9., Part 3, pp. 1304-1326.
Baird, D.J., Nelson, K.D., Knapp, J.H., Walters, J.J., and Brown, L.D., 1996, Crustal structure and evolution of the Trans-Hudson orogen: Results from seismic reflection profiling:
Tectonics, vol. 15, no. 2, pp. 416-426.
Bjorlie, P. F. and Anderson, S. B. , 1978, Stratigraphy and depositional setting of the Carrington Shale facies (Mississippian) of the Williston Basin: in Estelle, D. and Miller, R., eds. ,
Williston Basin Symposium: Montana Geological Society 24th Annual Conference, p. 165-176.
Bluemle, J. P., 1996, Oil and gas in North Dakota prior to 1951 - what preceded Iverson, North Dakota Geological Survey Newsletter, V. 23, No. 4, pp. 14-18.
Brown, D. L., and Brown, D. L., 1987, Wrench-style deformation and paleostructural influence on sedimentation in and around a cratonic basin, in Longman, M. W., ed., Williston Basin:
Anatomy of a cratonic oil province: Rocky Mountain Association of Geologists, p. 57-70.
Carlson, C.G., 1960, Stratigraphy of the Winnipeg and Deadwood Formations in North Dakota: North Dakota Geological Survey Bulletin 35, 145p.
Carlson, C.G., and Thompson, S.C., 1987, Stratigraphy of the Deadwood Formation and Winnipeg Group in the Williston Basin: in Longman, M. W., ed., Williston Basin: Anatomy of a cratonic
oil province: Rocky Mountain Association of Geologists, p. 71-82.
Carroll, W. K., 1979, Depositional environments and paragenetic porosity controls, upper Red River Formation, North Dakota: North Dakota Geological Survey Report of Investigation 66, 51 p.
Chimney, P. J., Treska, C. E., and Wolosin, C. A., 1992, Richardton/Taylor Fields - U.S.A., American Association of Petroleum Geologists Treatise of Petroleum Geology, Stratigraphic Traps III, pp. 421-445.
Clement, J. H., 1987, Cedar Creek: A significant paleotectonic feature of the Williston Basin, in Longman, M. W., ed., Williston Basin: Anatomy of a cratonic oil province: Rocky Mountain Association
of Geologists, p. 323-336.
Dean, K., 1982, Devonian Dawson Bay Formation in northwestern North Dakota: in Christopher, J. E. and Kaldi, J., eds., Fourth International Williston Basin Symposium Volume: Saskatchewan Geological Survey
Special Publication 6, p. 89-92.
Desch, J. B., Larsen, W. K., Lindsay, R. F., and Nettle, R. L., 1984, Enhanced oil recovery by CO2 miscible displacement in the Little Knife Field, Billings County, North Dakota: Journal Petroleum
Technology, v. 36, p. 1592-1602.
Dow, W.G., 1974, Application of oil correlation and source-rock data to exploration in the Williston Basin: American Association of Petroleum Geologists Bulletin, v. 58, p. 1253-1262.
Dumonceaux, G.M., 1984, Stratigraphy and depositional environments of the Three Forks Formation (Upper Devonian), Williston Basin, North Dakota: University of North Dakota Unpublished M. S. Thesis, 189 p.
Ehrets, J. R., and Kissling, D. L., 1985, Deposition, Diagenesis and Paleostructural control of Duperow and Birdbear (Nisku) reservoirs, Williston Basin, in Longman et al., eds., S. E. P. M.
Core Workshop No. 7, Golden, CO, pp. 183-216.
Ehrets, J. R., and Kissling, D. L., 1987, Winnipegosis platform margin and pinnacle reef reservoirs, northwestern North Dakota: in Fischer, D. W., ed., Fifth International Williston Basin
Symposium Core Workshop Volume: North Dakota Geological Survey Miscellaneous Series 69, p. 1-32.
Folsom, C. B., 1973, Petroleum and Natural Gas, in Mineral and Water Resources of North Dakota, North Dakota Geological Survey Bulletin 63, 99-119.
Foster, N. H., 1972, Ordovician System, Geologic atlas of the Rocky Mountain region, U.S.A.: in Rocky Mountain Association of Geologists, p. 76-85.
Fowler, C. M. R., and Nisbet, E. G., 1985, The subsidence of the Williston Basin, Canadian Journal of Earth Sciences, V. 22, No. 3, pp. 408-415.
Fuzesy, L., 1982, Petrology of Potash ore in the Esterhazy member of the Middle Devonian Prairie Evaporite in southeastern Saskatchewan, in Christopher, J. E. and Kaldi, J., eds .,
Fourth International Williston Basin Symposium Volume: Saskatchewan Geological Survey Special Publication 6, p. 67-73.
Gaston, L. D., Sherwin, D.F., and Allan, J. F. , 1964, Middle Devonian, in R. G. McCrossan and R. P. Glaister, eds., Geologic History of Western Canada, Alberta Society of Petroleum Geologists, p. 49-59.
Gerhard, L.C., Anderson, S.B., LeFever, J. A., Carlson, C.G., 1982, Geological development, origin, and energy mineral resources of the Williston Basin, North Dakota: American Association of
Petroleum Geologists Bulletin, v. 66, p.989-1020.
Gerhard, L. C., Anderson, S. B., and LeFever, J. A., 1987, Structural history of the Nesson anticline, in Longman, M. W., ed., Williston Basin: Anatomy of a cratonic oil province:
Rocky Mountain Association of Geologists, p. 337-353.
Green, A.G., Weber, W., Hajnal, Z., 1985, Evolution of Proterozoic terranes beneath the Williston Basin: Geology, v. 13, p.624-628.
Harris, S. B., Land, C. B. Jr., and McKeever, J. H., 1966, Mission Canyon stratigraphy, North Dakota, American Association of Petroleum Geologists Bulletin, v. 50, p. 2269-2276.
Heck, T. J. , 1979, Depositional environments and diagenesis of the Mississippian Bottineau interval (Lodgepole) in North Dakota: University of North Dakota Unpublished M. S. Thesis, 227p.
Heck, T. J., 1987, An overview of Dolphin field, Divide County, North Dakota, North Dakota Geological Survey Miscellaneous Series 70, 14 p.
Hendricks, M.L., 1988, Shallowing-upward cyclic carbonate reservoirs in the Lower Ratcliffe interval (Mississippian), Williams and McKenzie Counties, North Dakota: in
Goolsby, S.M. and Longman, M. W., eds., Occurrence and petrophysical properties of carbonate reservoirs in the Rocky Mountain region: Rocky Mountain Association of Geologists, p. 371-380.
Inden, R.F., Cluff, R. M., Byrnes, A.P., 1988, Reservoir geology and petrophysics of the Upper Interlake Group, Nesson anticline area, North Dakota:
in Goolsby, S.M. and Longman, M. W., eds., Occurrence and petrophysical properties of carbonate reservoirs in the Rocky Mountain region: Rocky Mountain Association of Geologists, p. 291-302.
Kendall, A. C., 1976, The Ordovician Carbonate Succession (Bighorn Group) of southeastern Saskatchewan, Department of Mineral Resources, Saskatchewan Geological Survey Bulletin 180, 185 p.
Kerr, S.D., Jr., 1988, Overview: Williston Basin carbonate reservoirs: in Goolsby, S.M. and Longman M . W. , eds. , Occurrence and petrophysical properties of carbonate reservoirs the Rocky
Mountain region: Rocky Mountain Association of Geologists, p. 251-274.
LeFever, J. A., 1991, History of oil production from the Bakken Formation, North Dakota, in, W. B. Hansen, ed., Montana geological Society 1991 Guidebook to Geology and Horizontal Drilling
of the Bakken Formation, Billings, MT, pp. 3-17.
LeFever, J. A., and Anderson, S.B., 1984, A little known carbonate reservoir within the Lower Lodgepole Formation, northwestern North Dakota: in Lorsong, J. A., and Wilson, M, eds., Oil and
Gas in n Saskatchewan: Saskatchewan Geological Survey Special Publication 7, p. 31-44.
LeFever, R. D., Thompson, S.C., Anderson, D.B., 1987, Earliest Paleozoic history of the Williston Basin in North Dakota: in Carlson, C. G. , and Christopher, J. E. , Fifth International
Williston Basin Symposium Volume: Saskatchewan Geological Society Special Publication 9, p. 22-37.
Levorson, A. I., 1967, Geology of Petroleum, 2nd Edition, W. H. Freeman and Company, San Francisco, 724 p.
Lindsay, R.F., 1988, Mission Canyon Formation reservoir characteristics in North Dakota: in Goolsby, S.M. and Longman, M. W., eds., Occurrence and petrophysical properties of carbonate reservoirs
in the Rocky Mountain region: Rocky Mountain Association of Geologists, p. 317-346.
LoBue, C.L., 1983, Depositional environments and diagenesis, Interlake Formation (Silurian), Williston Basin, North Dakota: University of North Dakota Unpublished M. S. Thesis, 233p.
Lobdell, F. K. , 1984, The Ashern Formation (Middle Devonian) in the Williston Basin, North Dakota: University of North Dakota Unpublished M. S. Thesis, 187p.
Lochman-Balk, C., 1972, Cambrian System, in Geologic atlas of the Rocky Mountain region, U.S.A.: Rocky Mountain Association of Geologists, p. 60-76.
Loeffler, P.T., 1982, Depositional environments and diagenesis, Birdbear Formation (Upper Devonian), Williston Basin, North Dakota: University of North Dakota Unpublished M. S. Thesis, 268p.
Longman, M. W., Fertal, T. G., and Glennie, J. S., 1983, Origin and geometry of Red River dolomite reservoirs, western Williston Basin, American Association of Petroleum Geologists Bulletin, v. 67, p. 744-771.
Megathan, E. R., 1987, Silurian Interlake Group: A sequence of cyclic marine and freshwater carbonate deposits in the central Williston Basin: in Fischer, D. W., ed., Fifth International
Williston Basin Symposium Core Workshop Volume: North Dakota Geological Survey Miscellaneous Series 69, p. 59-88.
Mitchum, Jr., R. M., Vail, P. R., and Thompson, S., 1977, Seismic stratigraphy and global changes of sea level, Part 2: The depositional sequence as a basic unit for stratigraphic analysis,
in, C. E. Payton, ed., Seismic Stratigraphy - application to hydrocarbon exploration, American Association of Petroleum Geologists Memoir 26, pp.53-62.
Oil & Gas Division, 1998, Oil in North Dakota; 1997 Production Statistics, Bismarck, ND, 624 p.
Oil & Gas Journal, 1999, Statistics, Oil & Gas Journal V. 97, No. 14, pp. 84-86.
Osadetz, K. G. and Snowdon, L. R., 1986, Speculation on the petroleum source rock potential of portions of the Lodgepole Formation (Mississippian) of southern Saskatchewan; in Current Research,
Part B, Geological Survey of Canada, Paper 86-1 B, p. 647-651.
Peterman, Z. E., and Goldich, S.S., 1982, Archean rocks of the Churchill basement, Williston Basin, North Dakota: In Christopher, J. E. and Kaldi, J., eds., Fourth international Williston Basin
Symposium Volume: Saskatchewan Geological Survey Special Publication 6, p. 11-12.
Pilatzke, R.H., Fischer, D. W., Pilatzke, C. L., 1987, Overview of Duperow (Devonian) production in the Williston Basin: in Longman, M. W., ed., Williston Basin: Anatomy of a cratonic oil
province: Rocky Mountain Association of Geologists, p. 423-432.
Price, L.C., Ging, T., Daws, T., Love, A., Pawlewicz, M., and Anders, D., 1984, Organic metamorphism in the Mississippian-Devonian Bakken shale North Dakota portion of the
Williston Basin: in Woodward, J., Meissner, F. S., and Clayton, J. L., eds. , Hydrocarbon source rocks of the greater Rocky Mountain region: Rocky Mountain Association of Geologists, p. 83-133.
Price, L.C., and J.A. LeFever, 1994, Dysfunctionalism in the Williston Basin: the Bakken/mid-Madison petroleum system: Bulletin of Canadian Petroleum Geology, vol. 42, no. 2, p. 187-219.
Hester, T.C., and Schmoker, J.W., 1985, Selected physical properties of the Bakken Formation, North Dakota and Montana part of the Williston Basin:
U.S. Geological Survey Oil and Gas Investigations Chart, OC-126, 1 sheet.
Sloss, L. L. , 1963, Sequences in the cratonic interior of North America: Geological Society of America Bulletin, v. 74, p. 93-114.
Sturm, S., 1982, Depositional environments and sandstone diagenesis in the Pennsylvanian Tyler Formation of southwestern North Dakota, in Christopher, J. E. and
Kaldi, J., eds., Fourth International Williston Basin Symposium, Saskatchewan Geological Society Special Publication No. 6, pp. 251-262.
Sturm, S., 1983, Depositional environments and sandstone diagenesis in the Tyler Formation (Pennsylvanian), southwestern North Dakota, North Dakota Geological
Survey Report of Investigation No. 76, 48 p.
Thompson, S. C., 1984, Depositional environments and history of the Winnipeg Group (Ordovician), Williston Basin, North Dakota, University of North Dakota, unpublished M. S. Thesis, 210 p.
Vail, P. R., Mitchum, Jr., R. M., and Thompson, S., 1977a, Seismic stratigraphy and global changes of sea level, Part 3: relative changes of sea level from coastal in, C. E.
Payton, ed., Seismic Stratigraphy- application to hydrocarbon exploration, American Association of Petroleum Geologists Memoir 26, pp.83-97.
Vail, P. R., Mitchum, Jr., R. M., and Thompson, S., 1977b, Seismic stratigraphy and global changes of sea level, Part 4: global cycles of relative changes of sea level, in,
C. E. Payton, ed., Seismic Stratigraphy - application to hydrocarbon exploration, American Association of Petroleum Geologists Memoir 26, pp. 83-97.
Voldseth, N. E., 1986, Coteau and Dale intervals of the Mississippian Mission Canyon Formation; Flaxton Field, Burke County, North Dakota, in Wyoming Geological
Association Rocky Mountain Oil and Gas Fields Symposium, p.
Webster, R. L. , 1984, Petroleum source rocks and stratigraphy of the Bakken Formation in North Dakota: in Woodward, J.,Meissner, F. S., and Clayton, J. L., eds.,
Hydrocarbon source rocks of the greater Rocky Mountain region: Rocky Mountain Association of Geologists, p. 5781.
Williams, J. A. , 1974, Characterization of oil types in the Williston Basin: American Association of Petroleum Geologists Bulletin, v. 58, p.1243-1252.
Wilson, J. L., 1967, Carbonate-evaporite cycles in lower Duperow Formation of the Williston Basin: Canadian Petroleum Geology Bulletin, v. 15, p. 230-312.