Click through the list for information on each resource.
Cement Rock
Cement is one of the basic components of concrete.
The terms cement and concrete are often used interchangeably. This can be confusing. Simply speaking, cement is a mixture of lime and silica which forms the binding component of concrete. There are several types of cement, but the most common variety is Portland. Portland Cement or Portland Grade Cement, is a cement material made by the grinding of a composite mixture of calcium, silicon, aluminum, iron, and other minor constituents, typically found in limestone and shale - or equivalent raw materials. The mixture is heated to incipient fusion (melting) in a rotary kiln, followed by fine grinding of the resulting clinker into a fine powder with the addition of gypsum (hydrous calcium sulfate) as a binding agent.
The Geological Survey undertook several studies of the feasibility of cement production in both eastern and western North Dakota during the 1890s, early 1900s, and again in the 1940s, 1950s, and 1960s (Babcock, 1901; Barry and Melstad, 1908; Powers, 1946; Hansen, 1953; Carlson, 1964; Anderson and Haraldson, 1968).
Several attempts have been made in North Dakota, starting in the early 1890s, to produce natural cement. Earle Babcock discovered lime-rich shales in the Niobrara Formation exposed at the surface in Pembina County. In 1899, Babcock, his brother Otto, and Webster Merrifield (President of the University of North Dakota from 1892-1909) incorporated the Pembina Portland Cement Company west of the town of Concrete in eastern Cavalier County. Babcock was State Geologist at that time and the potential for a conflict of interest existed with this arrangement. In 1909, it was reorganized as the Northern Cement and Plaster Company and produced bricklayer cement, hydraulic cement, cement plaster, and stucco (Folsom, 1980; Barry and Melstad, 1908). A zone of highly calcareous shale in the upper Niobrara Formation (Cretaceous) is present in scattered outcrops along the valley of the Tongue River in the vicinity of the cement plant. The calcareous shale was extracted through mine tunnels; approximately 900 feet of tunnels were sunk into outcrops along the valley of the Tongue River. The calcareous shale was baked in kilns at the plant, ground into a fine powder, and packaged. The natural cement produced at the plant had a difficult time competing with superior grades of Portland cement and ceased operation in 1909 as a result of problems in production and marketing (Grand Forks Herald, 1957). In 1955, a fire destroyed the main building and today the only evidence of the plant's existence are the foundations of a half dozen buildings, the base of the kilns, and scattered pieces of rusting equipment.
Despite the scientific studies carried out by the Geological Survey and the economic studies by the North Dakota Research Foundation, a Portland cement plant was never built in North Dakota. As a result, the calcareous rocks in North Dakota have not been mined for cement rock since the short-lived plant at Concrete closed in 1909.
However, there has been recent (ca. 2022) renewed interest from industry in the possibilities of developing the calcareous shales of the Niobrara Formation in northeastern North Dakota and other cement rocks in the state in order to reduce carbon emissions associated with traditional cement plant production.
Selected Resources for North Dakota Cement Rock
Anderson, F.J., 2005, Cement Rock Mineral Resources of the Shawnee-McCanna Area, North Dakota: North Dakota Geological Survey Geologic Investigation Nos. 22A, 22B, 22C, 22D, 22E
Anderson, F.J., 2006, Cement Rock Mineral Resources of the Niobrara Formation in Northeastern North Dakota: Department of Mineral Resources Geo News Newsletter, vol. 33, no. 1, p. 1-5.
Anderson, F.J., 2011, Niobrara Formation Cores in North Dakota: North Dakota Geological Survey Geologic Investigation No. 143, 1p. GI-143
Anderson, F.J., 2022, Investigations of the Niobrara Formation in Eastern North Dakota as Potential Cement Rock: Department of Mineral Resources Geo News Newsletter, vol. 49, no. 1, p. 8-10.
Anderson, S.B. and Haraldson, H.C., 1968, Cement-rock possibilities in Paleozoic rocks: North Dakota Geological Survey Report of Investigation No. 48, 62 p. RI-48
Carlson, C.G., The Niobrara Formation of eastern North Dakota: its possibilities for use as a cement rock: North Dakota Geological Survey Report of Investigation No. 41, 56 p. RI-41
Hansen, Miller, 1953, Geologic report on limestone deposits in Stark County and Hettinger County, North Dakota: North Dakota Geological Survey Report of Investigation No. 8, 33 p. RI-8
Murphy, E.C., 1994, Cement Rock in North Dakota, Department of Mineral Resources Geo News Newsletter, vol. 21, no. 4, p. 11-15.
Murphy, E.C., Hoganson, J.W., and Forsman, N.F., 1993, The Chadron, Brule, and Arikaree Formations in North Dakota: North Dakota Geological Survey Report of Investigation No. 96, 144 p. RI-96
Clay
The clays of North Dakota can be grouped into three general categories: bedrock clays, glacial clays, and lacustrine clays. Since three-fourths of North Dakota is covered by glacial sediments, glacial clays are the most abundant at or near the surface. However, the glacial clays have not been utilized very much over the years except for the early part of the twentieth century when they were used in the manufacture of brick. It is the bedrock clays, especially the kaolinitic-rich clays in the Golden Valley Formation that have proven to be the most useful. Aside from the lignite industry, the Golden Valley Formation has been one of the more utilized rock units exposed at the surface in western North Dakota.
The North Dakota Geological Survey (NDGS) has a long history of clay studies that go as far back as 1892. In 1892, Earle J. Babcock, Dean of the Chemistry Department and Mining Department at the University of North Dakota and State Geologist from 1895 to 1902, published the first article on the various types of clays and claystones in the state. The Bear Den Member was the focus of many of these early studies, called the “white fire clays” by Leonard (1906) and the “white clays” or “white high-grade clays” by Clapp and Babcock (1906).
The primary use of claystones in North Dakota has been for brickmaking. Bricks were produced as early as 1868 by soldiers who replaced log buildings with brick at Fort Ransom. Bricks were first produced for commercial use in North Dakota at Fargo in the 1870s. Brickmaking quickly spread through the Red River Valley and by 1885 plants were operating at Minto, Walhalla, Grafton, and four plants in Grand Forks. More than thirty brick plants operated in North Dakota between the 1870s and 1920s. Most of the plants produced common brick, but pressed brick, facing brick, and fire brick were also produced at a few sites. Throughout North Dakota, bricks were made from clays of different geologic origins. One brick plant at Mayo used Cretaceous shales while brick plants at Kenmare, Donnybrook, Burlington, Velva, New Salem, Hebron, Dickinson, and Wilton used claystones from the Fort Union Group. Brick plants at Bismarck, Rolla, and Richardton produced bricks from till whereas glacial Lake Agassiz clay was used at four plants at Grand Forks, two at Fargo, and one each at Drayton and Abercrombie. Another plant at Omemee used glaciolacustrine clay from Lake Souris. Plants at Mandan, Williston, Minot, and Hillsboro used alluvial clays to make bricks (Clapp and Babcock, 1906). The Hebron Brick Company began manufacturing bricks using claystone from the Bear Den Member in 1904. The Dickinson Fire and Pressed Brick Company began mining the Bear Den Member along the Heart River south of Dickinson in the early 1900s. That facility closed in the late 1930s. In the 1960s, the Dickinson Clay Products Company produced ceramic sewer pipe and tiles from Bear Den Member claystones but the plant was short-lived due to competition from plastic sewer pipe. Claystone from this stratigraphic horizon as well as the Rhame Bed have also been used to make pottery.
Through the diligent efforts of Earle Babcock, Charles Clapp, William Budge, and Oscar Manz, clays and claystones in North Dakota have been identified, chemically analyzed, and mechanically tested for their usefulness in many manufactured products. North Dakota clays have been used for a variety of purposes including brick making, pottery, sewer pipe, drain tile, lightweight aggregate, soaps and cleansers, kitty litter, floor adsorption material, and as a source of alumina. Geologists over the years have also investigated clay deposits for their potential as bleaching clays, to produce electrical insulators, re-refining used lubrication oils, and as cement rock.
Selected References for North Dakota Clay
Babcock, E.J., 1906, The uses and value of North Dakota clays, in the Fourth Biennial Report of the North Dakota Geological Survey, p. 191-244. BR-4
Budge, W.E., 1932, Report of Surface of Tertiary clays in southwestern North Dakota: University of North Dakota College of Engineering and Mines and Mining Experiments, p. 21-38.
Budge, W.E., 1936, The Bentonites of North Dakota: University of North Dakota School of Mines, 17 p.
Clapp, C.H. and Babcock, E.J., 1906, Clay and its properties with special reference to North Dakota clays, in the Fourth Biennial Report of the North Dakota Geological Survey, p. 9-62. BR-4
Clapp, C.H. and Babcock, E.J., 1906, Economic Geology of North Dakota clays, in the Fourth Biennial Report of the North Dakota Geological Survey, p. 95-190. BR-4
Clark, F.F., 1948, Southwestern North Dakota clay deposits Stark, Slope, and Billings counties, North Dakota: United States Bureau of Mines Report of Investigations No. 4219, 32 p.
Fritz, A., 1998, North Dakota Clay: Department of Mineral Resources Geo News Newsletter, vol. 25, no. 1, p. 13-16.
Hansen, M., 1959, Clays of North Dakota as a potential source of alumina: North Dakota Geological Survey Report of Investigation No. 33, 15 p. RI-33
Manz, O.E., 1953, Investigation of some North Dakota clays and shales: North Dakota Geological Survey Report of Investigation No. 13, 36 p. RI-13
Manz, O.E., 1954, Investigation of lightweight aggregate possibilities of some North Dakota clays and shales: North Dakota Geological Survey Report of Investigation No. 17, 48 p. RI-17
Manz, O.E., 1956, Investigation of Lake Agassiz clay deposits: North Dakota Geological Survey Report of Investigation No. 27, 34 p. RI-27
Manz, O.E., 1973, Clay and lightweight aggregate in Mineral and Water Resources of North Dakota: North Dakota Geological Survey Bulletin 63, p. 122-129.
Murphy, E.C., 1995, North Dakota clays, a historical review of clay utilization in North Dakota: North Dakota Geological Survey Miscellaneous Series No. 79, 18 p. MS-79
Coal
The recorded history of lignite mining in North Dakota goes back to 1873, west of Bismarck. Many of the earliest attempts at mining lignite coal were failures, but a substantial number of mines, opened throughout the 1880s and 1890s, were successful. Small mines sprung up along the main routes of transportation in western North Dakota. By 1900, the lignite mining industry had a secure foundation in the state of North Dakota. At least 73 mines were operating in the state by 1900. Many of these one-man operations were small, surface mines that removed coal from the face of the outcrop. They were called wagon mines because area farmers and ranchers would often bring their own wagons to the site to be filled with coal. Still, other mines were large underground operations employing hundreds of men with underground workings extending for thousands of feet.
In the spring of 1901, Clement A. Lounsberry, editor of The Record, a widely read magazine of the North Dakota Historical Society out of Fargo, declared to the people of North Dakota that, "vast fields of lignite coal were stored up under the clay and loam of the North Dakota soil..." and with supreme confidence, he proclaimed that, "...it would be no exaggeration to say that there is enough of it to warm the entire population of the United States for ten thousand years." Although a dramatic statement by Lounsberry, it was indicative of the exuberant attitude prevalent among coal mine operators, land developers, literary men, and state boosters.
All of the economic or mineable coal in North Dakota is found within the lower Fort Union Group in western and central North Dakota and is Paleocene in age. That is to say that the peat from which this lignite formed was deposited in swamps 55 to 65 million years ago. Judging from the number and the lateral extent of some of these beds, swamps covered a large portion of the western half of North Dakota during this time period. We are not able to determine what was occurring in the eastern part of the state at this time because rocks of this age are missing.
By the early 1920s, approximately 250 mines were operating in the state, with an equal number of underground and surface strip mines. By 1929, horses and mules had been replaced by huge steam shovels, undercutting machines, drills, and narrow-gauge railroads. The new technology also reduced the strenuous labors of men and elevated them to the position of controlling the power machines. Many of the large mines, both underground and strip, were totally mechanized by 1929. In 1939, the number of mines operating in the State reached a record high of 306, with over half of these being strip (open pit) mines. The advent of the steam shovel increased the profitability of the surface mines and the last underground mine ceased operation in 1966.
Underground mines have caused problems in many areas where the collapse of the mine tunnels has created sinkholes at the surface. The North Dakota Public Service Commission has spent considerable time and money attempting to rectify this sinkhole problem by digging out the old mine workings where they are close to the surface and by pumping grout into the deeper workings to stabilize them. In several areas of the state (Dickinson, Minot, and Beulah), these underground workings are beneath highways or county roads and have been grouted to stabilize the road. It is interesting to note that as early as the 1920s, geologists for the North Dakota Geological Survey were noting the collapse features of underground mines and warning that these underground workings would be posing an increasing problem in the future.
The 1962 Democratic State Platform proclaimed that North Dakota would become the "power hub of the nation." Accordingly, in 1966, the first significant coal-fired generating station began operations at Stanton, North Dakota. Known as the Leland Olds Station, it was built by Basin Electric Power Cooperative, Inc., a consortium of rural energy companies. United Power Association constructed a second plant at Stanton in 1968. A third generating station, named after Senator Milton R. Young and built by Minnkota Power Cooperative, Inc., at Center, North Dakota, was dedicated in 1970. As the United States entered the energy crisis years during the Ford and Carter Administrations - a crisis precipitated in great measure by the oil embargo of 1973, the state and the nation turned to their vast coal supplies as a source of electrical power. From 1975 to 1981, several new generating stations and units were constructed in North Dakota, with each unit producing three billion kilowatt hours annually. The new facilities, fired by lignite from nearby mines, included the Leland Olds Station #2 in 1975, the Coal Creek Plants #1 (1979) and #2 (1980), the Coyote Station in 1981 and the Square Butte Creek Plant, also known as the Milton R. Young Station #2 in 1977.
With the establishment of these electrical generation plants, the production of lignite increased significantly. Between 1966 and 1970, the amount of lignite mined each year increased from 3,067,119 tons to 5,001,828 tons. During the decade of the 1970s, the production of coal more than tripled. By 1981, the lignite production level in North Dakota reached 17,613,742 tons.
In addition to the existing facilities, the twin units of the Antelope Valley Plant near Beulah became operational in 1984 and 1986. The gasification of North Dakota's soft coal also became a reality with North American Natural Gas Company's Beulah plant, which began operating in 1984.
The giant draglines and twin smokestacks, symbols of the mining and energy industries, changed the landscape of western North Dakota during the 1970s. Today they are part of it.
Selected References for North Dakota Coal
Babcock, E.J., 1901, Report of the North Dakota Geological Survey; Coal: North Dakota Geological Survey First Biennial Report, 103 p. BR-1
Brant, R.A., 1953, Lignite resources of North Dakota: United States Geological Survey Circular 226, 78 p.
Groenewold, G.H., Hemish, L.A., Cherry, J.A., Rehm, B.W., Meyer, G.N., and Winczewski, L.M., 1979, The geology and geohydrology of the Knife River Basin and adjacent areas of west-central North Dakota: North Dakota Geological Survey Report of Investigation No. 64, 402 p. RI-64
Leonard, A.G., Babcock, E.J., and Dove, L.P., 1925, The lignite deposits of North Dakota: North Dakota Geological Survey Bulletin No. 4, 240 p. B-4
Moran, S.R., Cherry, J.A., Fritz, P., Peterson, W.M., Somerville, M.H., Stancel, S.A., and Ulmer, J.H., 1978, Geology, groundwater hydrology, and hydrogeochemistry of a proposed surface mine and lignite gasification plant site near Dunn Center, North Dakota: North Dakota Geological Survey Report of Investigation No. 61, 263 p. RI-61
Murphy, E.C., 1998, The Coteau lignite bed in north-central North Dakota: North Dakota Geological Survey Open-File Report 98-2, 11p. OF-98-2
Murphy, E.C., 2001, Strippable lignite deposits of North Dakota: North Dakota Geological Survey Miscellaneous Map no. 34, 1:1,000,000 scale. MM-34
Murphy, E.C., 2002, The Coal Butte Mine - A Long Crawl for Coal: Department of Mineral Resources Geo News Newsletter, vol. 29, no. 1, p. 1-3.
Murphy, E.C., and Goven, G.E., 1998, The coalbed methane potential of North Dakota lignites: North Dakota Geological Survey Open-File Report 98-1, 38 p. OF-98-1
Oihus, C.A., 1983, A history of coal mining in North Dakota 1873-1982: North Dakota Geological Survey Educational Series No. 15, 100 p. ED-15
Pollard, B.C., Smith, J.B., and Knox, C.C., 1 972, Strippable lignite reserves of North Dakota: United States Bureau of Mines Information Circular 8537, 37 p.
Geothermal Energy
Geothermal energy is a renewable resource capable of producing an uninterrupted supply of electrical power and heat. In stable sedimentary basins, low-temperature energy (<40°C, < 100°F) is extracted from the shallow subsurface (~8-400 feet) for use in domestic and commercial heating and cooling systems. Historically, deeper, hotter resources in these regions have not been developed because they typically lack one or more of the essential requirements that make high-temperature geothermal resources technically and economically viable. However, as the nation explores ways to reduce its dependency on foreign energy sources and also begins to look more closely at renewable energy, accessing deep geothermal energy resources, particularly via existing oil and gas wells, is attracting a great deal of interest.
Conventional methods of electricity generation using geothermal energy rely on hot (> 100°C) relatively shallow (<3,000 m [10,000 feet]), easily developed hydrothermal resources. Generally associated with active plate boundaries and/or volcanism, these high-grade hydrothermal systems are characterized by high thermal gradients, and highly fractured, porous reservoir rocks through which natural waters or steam can freely circulate. Large-scale, cost-effective electric power generation usually requires fluid temperatures above 150°C but smaller systems based on standard binary-cycle technology are capable of producing electricity using geothermal fluids at temperatures as low as 100°C.
Natural sources of high-grade hydrothermal energy are geographically limited. In the U.S. they are restricted to the western states and currently represent less than 1% of the nation’s electrical power generating capacity. Yet the amount of heat at depths less than 10,000 m (30,000 feet) below the surface of the continental U.S. is substantial. By replicating natural hydrothermal conditions, it is possible, in some regions, to turn this heat into an economically viable resource.
Enhanced (or engineered) geothermal systems (EGS) are engineered reservoirs designed to produce energy as heat or electricity from geothermal resources that are otherwise not economical due to lack of water and/or permeability (U.S. Department of Energy, 2008). EGS technology uses adaptations of techniques developed in the oil and gas, and mining industries to fracture hot, low-porosity rocks in the deep subsurface and extract the heat with water via a system of injection and production wells. With infrastructures already in place and the abundance of horizontally drilled and/or artificially stimulated wells, hydrocarbon fields are prime candidates for the application of EGS technology. Of particular interest are those wells regarded as marginal or unproductive because they produce too much water. Geothermal waters that are coproduced with oil and gas are an expensive waste product that in North Dakota must be disposed of by re-injection into the subsurface. If sufficiently hot (>100°-150°C) and available in sufficient quantity, however, these waters may be capable of generating cost-effective electricity (McKenna and others, 2005).
All geothermal (geoexchange) systems have three main components: (i) a means of transferring heat to and from the subsurface, (ii) a heating and cooling device (heat pump), and (iii) a heating and cooling distribution system.
Heat is extracted from, or transferred to, the subsurface via a series of high-density polyethylene (HDPE) loops through which a fluid is circulated. This fluid may be water or an aqueous solution of a pre-approved heat transfer material such as propylene glycol or denatured ethanol. Loop systems are classified as either open or closed, and those loops can be configured in one of three ways: vertically, horizontally, or in a pond/lake. The type chosen depends on the available land area, and the soil and rock type at the installation site. These factors will help determine the most economical choice for the installation of the ground loop.
In an open-loop geothermal system, water is pumped directly from an aquifer via one or more wells into the building where it passes through a heat pump before being returned to the aquifer by a separate (discharge) well or released into a nearby lake or stream. However, despite their relative simplicity, open-loop geothermal systems are not as popular as the closed-loop variety. This is largely due to the fact that they require an abundant, clean groundwater supply and in some states, including North Dakota, may be subject to additional regulatory control.
In closed-loop systems, the heat transfer fluid flows through a continuous, closed circuit of loops, which are installed in the ground in either a vertical or horizontal configuration or underwater in a lake or pond. Most geothermal systems in North Dakota are vertical closed-loop systems. The construction of a vertical closed-loop geothermal system requires the drilling of a series of holes, about four inches in diameter, spaced 15 to 20 feet apart, and ranging in number from as few as four to several hundred, depending on the size of the building, and between 100 and 400 feet deep (typically 150-200 feet in North Dakota). Two 1¼-inch HDPE pipes, connected by a u-tube, are inserted into each well and cemented in place with a thermally conductive grout. The loops are connected in a series by a horizontal manifold, which carries the heat transfer fluid to and from the heat pump. Once the loops are connected, the ground surface is leveled and smoothed. Very often it is grassed over or paved, leaving no visible evidence of the well field beneath.
Horizontal closed loop systems are constructed in a similar way to vertical systems except that the loops are laid horizontally, or coiled into a “slinky” shape if space is limited, in trenches about six feet deep. The “slinky” is also the loop design of choice for pond/lake systems where the loop field is installed underwater instead of in the ground. A minimum annual water depth of six feet is required for these systems to function efficiently and needless to say, they are not common in North Dakota.
The heart of any geoexchange system is the geothermal heat pump. Also known as ground source heat pumps these highly efficient devices may be viewed very simply as a sort of two-way air conditioner. Their principles of operation are the same as their objective – to move heat from one place to another. In summer, heat is withdrawn from the building and discharged into the ground via the circulating heat transfer fluid. In winter, the process is reversed, and heat is extracted from the ground. However, unlike a conventional furnace, which heats air drawn from outside, a geothermal heat pump extracts heat from a fluid that is already relatively warm – a far more cost-effective method.
Besides a power source, all heat pumps consist of a compressor, an expansion valve, a condenser, and an evaporator, which are connected via a system of pipes and coils filled with refrigerant. Geothermal heat pumps have an additional component - a reversing valve - which allows the refrigerant to flow through the system in both directions (heating vs. cooling mode).
Inside the building, the geothermal heat pump quietly goes about its business. Because they have few moving parts and do not have external condensing units like air conditioners, geothermal heat pumps are durable, very reliable, and produce little noise. Typically, they can be expected to last at least 20 years.
Since 1984, geothermal heating and cooling systems have been regulated by the North Dakota Geological Survey under Chapters 38-19 and 43-02-07 of the North Dakota Century and Administrative Codes respectively. The regulatory program was introduced to ensure that geothermal systems are designed and constructed according to certain standards and do not pose any kind of environmental hazard such as surface or groundwater contamination.
-Geothermal Energy: Another Alternative by Lorraine A. Manz, NDGS
Selected References for Geothermal Energy
Kruger, N.W., 2024, Getting Into Hot Water: Department of Mineral Resources Geo News Newsletter, vol. 51, no. 1, p. 6-7.
Kruger, N.W., 2024, Promoting Geothermal Heat Pumps: Department of Mineral Resources Geo News Newsletter, vol. 51, no. 2, p. 12-13.
Manz, L.A., 2018, Geothermal Energy Update: Department of Mineral Resources Geo News Newsletter, vol. 45, no. 1, p. 20-22.
Manz, L.A., 2012, Geothermal Update: Department of Mineral Resources Geo News Newsletter, vol. 39, no. 1, p. 19-20.
Manz, L.A., 2011, Geothermal Energy Update: Another First For North Dakota: Department of Mineral Resources Geo News Newsletter, vol. 38, no. 1, p. 5-6.
Manz, L.A., 2007, Geothermal Energy Update: Another Alternative: Department of Mineral Resources Geo News Newsletter, vol. 34, no. 1, p. 1-6.
Murphy, E.C., 1990, Geothermal Production in North Dakota: Department of Mineral Resources Geo News Newsletter, no. 2, p. 14-17.
Petroleum
Hydrocarbons have been produced in North Dakota for many years. The oldest natural gas, or methane, production was non-commercial production reported from a Dakota Sandstone water-well near Edgeley in 1892 (Anderson and Eastwood, 1968). Later, shallow gas was discovered in Bottineau County in sufficient quantity to supply the towns of Mohall, Westhope, Lansford, and some small towns and farmsteads during the early 1900s but, by 1920, the produced volume had decreased so much that only a few farmsteads could still be supplied. The oldest commercial hydrocarbon production in North Dakota was established in 1929 when the Cedar Creek gas field was extended into Bowman County from Montana. Production is from the Cretaceous Eagle and Judith River sandstones. Development of the gas continued sporadically with wells being completed in the mid-1940s and the late 1970s to early 1980s. Less than 100 gas wells were drilled on the Cedar Creek anticline in North Dakota.
Oil is the most important hydrocarbon produced in the state, but oil was not discovered until 1951 when Amerada Hess Corporation completed the Clarence Iverson #1 on the Nesson anticline. Since then, oil exploration has been nearly continuous in the state. The discovery well was completed in the Silurian Interlake Formation but subsequent development on the anticline focused on the Mississippian Madison Group. Annual oil production peaked in 1984 at more than 52,000,000 barrels of oil but a decline followed. The decline was temporarily reversed in the late 1990s, so much so that daily oil production briefly rose above 100,000 barrels of oil per day for the first time since 1991 in late 1997.
Bakken-Three Forks production has expanded over the past two decades from a handful of scattered vertical and horizontal wells to the most prominent oil play in the history of the Williston Basin. Exploration and development of the Bakken Petroleum system as a modern unconventional oil play (horizontal drilling coupled with hydraulic fracture well completions) began in the mid-2000s within western North Dakota. While initial exploration and development focused on the Middle Bakken reservoir, the upper Three Forks evolved into a second primary reservoir during the late 2000s. To date, more than 17,500 wells have been drilled and completed within the Middle Bakken and upper Three Forks Formations (USGS, 2021).
Horizontal drilling in the middle Three Forks began in late 2012 followed by initial well completions and production in early 2013. By the end of 2020, more than 250 horizontal middle Three Forks wells had been drilled and completed with combined cumulative production of more than 57 million barrels of oil and 120 billion cubic feet of gas. Even though fluctuating oil prices cause drilling and production activity to vary year to year, the Bakken-Three Forks play has created thousands of in-state jobs which has bolstered North Dakota’s population and economy.
Crude oil is a naturally occurring complex liquid hydrocarbon whose main chemical components are hydrogen and carbon. Crude oils contain many other elements and compounds, like sulfur, nitrogen, iron, or sodium chloride (Levorson, 1967). North Dakota crude oils are generally paraffinic-based (Folsom, 1973), that is they belong to the paraffinic or methane series. Most of the crude oils produced in the state range from 19 to 55 degrees of API gravity. The Third Edition of the Glossary of Geology defines a paraffin series as a homologous series of saturated aliphatic hydrocarbons, empirical formula CnH2n+2, of which methane is the lowest and representative member, followed by ethane, propane, the butanes, etc. Some paraffinic crude oils, like those produced from the Pennsylvanian Tyler Formation, contain so much paraffin that a solid, waxy material will precipitate from the crude as its temperature decreases. This paraffin can be a valuable component of the crude and is most commonly found in stores as petroleum jelly. Crude oil is measured in standard barrels and one barrel of oil contains 42 U.S. gallons.
Most of the crude oils in North Dakota also contain some natural gas. At reservoir temperatures and pressures, the gas is dissolved in the oil but, when the oil is brought to the surface, the oil and gas separate into liquid and gas phases. When all the gas in the reservoir is dissolved in the oil at reservoir conditions it is called associated gas, and the oil is undersaturated with natural gas. If there is more natural gas than the crude oil can contain, then the oil is saturated. In this situation, two phases of hydrocarbons can form in the reservoir, a gas phase, or gas cap, which forms in the structurally highest part of the reservoir, and a liquid oil phase that is found underneath it. Both saturated and undersaturated reservoirs are usually considered to be oil reservoirs. If only gas is present in the reservoir, then it is a gas reservoir. Most reservoirs in North Dakota are undersaturated oil reservoirs. Gas reservoirs are rare in North Dakota as are reservoirs with a free gas cap.
Most of the natural gas found in North Dakota is "wet gas", it contains hydrocarbons that are liquid under pressure but a gas at surface pressures. Some of these wet gases are propane and butane and they are a valuable by-product to the oil producers as they are usually sold separately from the crude oil and methane. Natural gas is sold in standard cubic feet measured at 60° F and 14.73 psi.
Once a well is on production, the oil and natural gas are separated at the well site. The oil is transported by either a truck or by pipeline to a refinery. The refinery at Mandan is the only operating refinery in North Dakota and it has a daily capacity of around 60,000 barrels of crude per day. The remaining crude oil is transported out of the state to other refineries by pipeline.
Produced natural gas is either used at location, flared at location, or pipelined to a gas processing plant. Natural gas is often used to operate equipment, like pumps or treaters, at the well site. It can be a significant savings over running power lines and buying the electricity to run the equipment. If, after lease use, the volume of gas produced is too small to justify the expense of building a pipeline to the well site, the gas is flared, or burnt, at location. Pipelines are expensive to build, costing tens of thousands of dollars per mile to build. To justify the expense, estimates of the amount of gas contained in a reservoir must be weighed against the distance, and thus cost, to the nearest point where a hook-up to the natural gas gathering system can be made. Oil companies drill wells to make money and if the economics aren't right, no pipeline will be built. When enough gas is produced to justify the cost, a pipeline is built to the well and the gas is sold. The gas goes from the well to one of the nine gas processing plants in North Dakota where gases like butane and propane are separated from the methane. The methane is then put into the regional gas pipeline system for sale and the various other wet gases are sold.
Hydrocarbon production is limited to seventeen counties in western North Dakota (Fig. 4). Some possible reasons for the limited distribution of oil and gas are: absent or immature source rocks, potential reservoir rocks have either been eroded off or weren't deposited, the migration paths of the hydrocarbons did not cross any other counties, or hydrocarbons are present but have not yet been discovered.
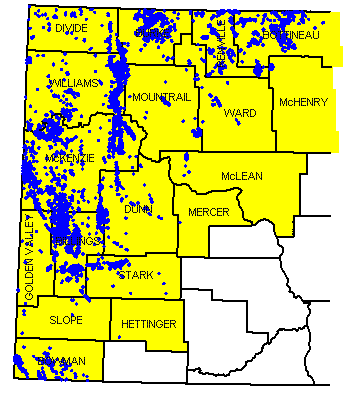
Figure 4. North Dakota oil producing counties (yellow) with the producing wells (blue).
General Geologic Setting
The Williston Basin is a large, roughly circular depression on the North American Craton covering several hundred thousand square miles across parts of North and South Dakota, Montana, and the Canadian provinces of Manitoba and Saskatchewan (Fig. 5). The Williston Basin began to subside during the Ordovician Period (Fig. 6), around 495 million years ago and it underwent episodic subsidence throughout the rest of the Phanerozoic Eon. The Phanerozoic Eon, that part of geologic time during which life was abundant, extends from approximately 600 million years ago to the present. Although the Williston Basin was subsiding, marine sediments were not deposited in it continuously. The basin contains an unusually complete rock record compared to many basins, but even in the Williston Basin erosion dominated a significant amount of the Phanerozoic Era (Fig. 7). However, some rocks from each Phanerozoic period are preserved in the basin. These strata record several cycles of marine transgressions that filled the basin, followed by marine regressions that drained the basin. Sloss (1963) defined six inter-regional rock-stratigraphic units that were traceable across the North American craton and named them sequences. One of the example areas Sloss used when he first proposed the sequence concept was the Williston Basin. He divided the Phanerozoic Era into six sequences which, in ascending order, are the Sauk, Tippecanoe, Kaskaskia, Absaroka, Zuni, and Tejas (Fig. 7).
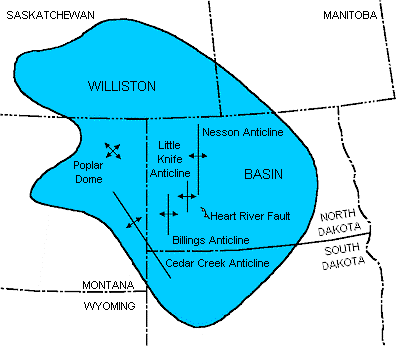
Figure 5. Extent of the Williston Basin with major North Dakota structures shown.
Figure 6. Generalized stratigraphic column for the Williston Basin with gas-producing horizons shown in red and oil-producing horizons shown in blue.
More recently, Sloss' sequence concept changed to a depositional sequence concept that is based upon the identification of sequences on seismic data (Mitchum, Vail, and Thompson, 1977).
The study of depositional sequences on seismic data has become known as seismic stratigraphy and is used worldwide as a major tool in exploration for oil and natural gas. Vail, Mitchum, and Thompson (1977a; 1977b) also recognized that depositional sequences record regional relative sea level changes which, if coincident with relative changes in many regions, are probably of global extent. They noted three orders of cycles and identified a number of cycles. They identified two first-order cycles, also called super cycles, that were 200-300 million years long and fourteen second-order cycles that lasted for 10 to 80 million years (Fig. 7). They also identified more than 80 third-order cycles, each lasting from 1 to 10 million years, but did not consider the pre-Jurassic cycles to be of global extent as the data came largely from North America.
Most of the hydrocarbons are produced from rocks deposited during the Paleozoic Era (Fig. 6) although some Mesozoic strata are productive. Since the initial discovery, the Madison Group (Mississippian) has produced the most oil, but significant volumes have also been found in the Red River (Ordovician) and Duperow (Devonian) formations (Table 1). Sandstones of the Spearfish Formation (Triassic) produce oil in north-central North Dakota and Eagle and Judith River Sandstones (Cretaceous) produce natural gas in southwestern North Dakota. Natural gas had been produced from other Cretaceous sands in Dickey and LaMoure counties and from glacial drift in Bottineau County, but none is produced from these strata now.
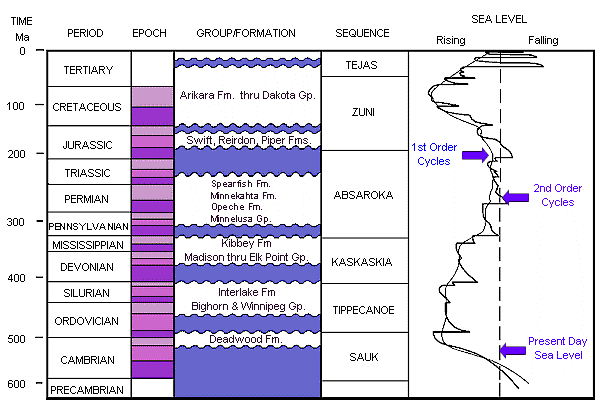
Figure 7. Time-stratigraphic column of the North Dakota Williston Basin with the First and Second Order sea level curves of Vail, et al. (Modified from Fowler and Nisbet, 1985).
Structural Geology
The Williston Basin, compared to many basins worldwide, is a structurally simple basin. It is roughly circular, deepest in its center, and the strata become both shallower and thinner towards its margins. It is a large basin, covering approximately 150,000 square miles over parts of North Dakota, South Dakota, and Montana and parts of the adjacent Canadian provinces of Saskatchewan and Manitoba. The basins deepest point is thought to be near Williston, ND where the Precambrian surface is more than 16,000 feet below the surface.
The early structural history of the basin, from the deposition and/or emplacement of the oldest rocks, is poorly understood. The earliest rocks are difficult to study because the Lower Phanerozoic and Precambrian rocks are not exposed at the surface in North Dakota and only a few wells have penetrated these rocks. Our present understanding of the early geologic history of the basin is pieced together from outcrops in adjacent states and provinces, from seismic data, and from the limited well data.
Precambrian rocks under North Dakota can be placed into one of three geologic provinces, the Superior Craton, the Wyoming Craton, or the Trans-Hudson orogen (Fig. 8). The Superior Craton underlies the eastern half of North Dakota and is a part of the North American Canadian Shield. The Superior Craton is Archean in age and consists mainly of granites and greenstones that were emplaced between 2.75-2.6 billion years ago. Underlying eastern Montana, and possibly extending into western North Dakota, is the Wyoming Craton (Archean). Rocks of the Wyoming Province are gneissic, or banded to lenticular feldspar and quartz-rich rocks, and are approximately the same age as rocks from the Superior Craton. Between these two cratons, under most of western North Dakota, lies the Trans-Hudson orogen, which is composed of oceanic material from an early rifting event within the Superior Craton. A later collision between the Superior craton and the Wyoming and/or Churchill cratons accreted volcanic island-arc material to the orogen. (Green et al, 1985). Rocks from the island-arc complexes are between 1.95 and 1.85 billion years old, or Early Proterozoic in age, while the oceanic material apparently is older, but still Proterozoic in age.
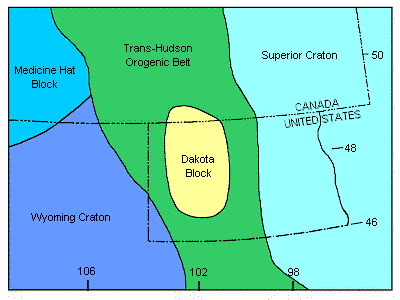
Figure 8. Structural elements in the vicinity of the Williston Basin (Modified from Green et al, 1985).
The internal structural geology of the two provinces and the orogen can be very complex, as are the structural relationships between them. Our limited ability to either sample the rocks or to seismically image them restricts our ability to determine how the Williston Basin formed. The early structural history of the basin has had an impact on later Phanerozoic deposition, but the extent of that impact is not always known. Although subsurface data is limited, in Beaver Lodge Field on the Nesson anticline, it can be demonstrated from well control that within a few miles, there are hundreds of feet of topographic relief on the Precambrian surface (Fig. 9). The relief on the basement surface is either an erosional remnant of a granite knob or is a horst block formed by movement along vertical to near-vertical faults.
Most of the structural deformation during the Phanerozoic Eon in North Dakota probably resulted from the subsidence of the Williston Basin. The main evidences of structural deformation in the basin are folding and faulting. The best evidence of folding in North Dakota is the anticlinal and synclinal structures that were formed. Some of these structures, like the Nesson, Cedar Creek, Little Knife, and Billings anticlines, produce oil (Fig. 5). Many other unnamed anticlinal structures exist in the basin, but only some of them have been proven to be oil-producing.
The other primary evidence of structural deformation is faulting, and faults are less well documented in North Dakota. Some faults, like those on the west flank of the Cedar Creek anticline (Clement, 1987) and the Heart River fault (Chimney et al., 1992) are visible on seismic. Clement (1987) shows faults to be steeply dipping, almost vertical, and reports that faults along the Cedar Creek anticline have undergone recurrent near-vertical and wrench movements. He also reports that the displacement direction along some of these faults changed over time. Faults have been inferred elsewhere, but well control is insufficient to prove their existence and/or the fault's displacement is too small to be imaged on seismic data. The inferred faults probably have similar characteristics to the larger faults on the Cedar Creek anticline, that is they dip nearly vertically, may have a component of wrenching, have undergone recurrent movements, and the direction and magnitude of displacement has changed over time. However, it is unlikely that most faults will be proven to exist because the near-vertical dip makes them difficult to intersect or image.
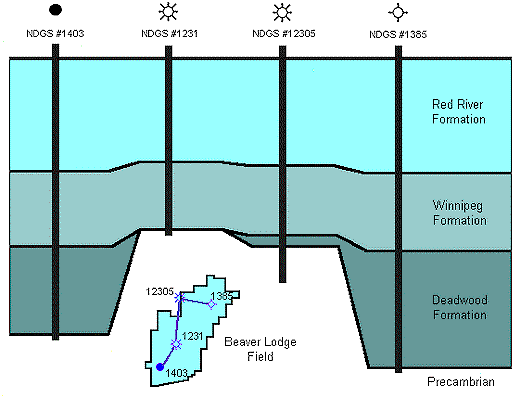
Figure 9. Cross section across Beaver Lodge Field of the Ordovician to Precambrian section. Note the relief on the Precambrian surface and the missing Deadwood section over the Precambrian high.
Features that are frequently interpreted to be present in the Williston Basin are lineaments. A lineament is the surface expression of a basement block boundary or fault (Brown and Brown, 1987). Tectonic stresses in the Williston Basin have changed over time and these stresses may have been relieved through vertical and/or lateral movement along pre-existing zones of weakness like a fault. The magnitude of individual displacements may not have been great, but the displacement was at least sufficient to have affected local deposition and erosion patterns. These local patterns often control where oil is trapped.
Stratigraphy and Petroleum Characteristics
Basement Rocks (Precambrian)
Basement is the crust of the earth extending from the base of sedimentary cover down to the Mohorovicic discontinuity, or all Precambrian rocks. Phanerozoic deposition in the Williston Basin began on a surface of weathered basement rocks.
The Precambrian is subdivided into two eons. The older is the Archeozoic Eon, from between 4 to 2.5 billion years while the younger, Proterozoic Eon occurred between 2.5 to 0.670 billion years. The geology of the Precambrian rocks underlying the Williston Basin is complex, consisting of many juxtaposed, fault-bounded lithostructural domains (Peterman and Goldich, 1982). Green et al (1985) suggested that the basement rocks in western North Dakota formed in an orogenic belt, or linear deformed area, called the Trans-Hudson orogen that lies between the Archean Superior and Wyoming Provinces (Fig. 8). More recently, Baird and others (1996) reported another, previously unknown block under western North Dakota which they named the Dakota block. They interpreted the block to be an Archean continental fragment because of similarities in its reflection geometry to Archean crustal material exposed in the Glennie domain in Canada. In general, basement rocks do not produce oil in North Dakota, but one well in Newporte Field did produce oil from fractured Precambrian rocks.
Sauk Sequence (Cambrian-Lower Ordovician)
The Upper Cambrian Deadwood Formation records both the earliest Phanerozoic sedimentation and the beginning of the Sauk Sequence in the Williston Basin (Fig. 6). The Cambrian sea transgressed eastward into an embayment on the edge of the Cordilleran shelf (Carlson, 1960; Lochman-Balk, 1972), and deposited siliciclastic sediments, sands and shales, as the dominant sediment type in North Dakota. During Lower Ordovician, carbonate sediments began to be deposited in the center of the basin, which was now formed and had begun to subside (LeFever et al., 1987). The Cambrian Deadwood Formation produces oil along the Nesson anticline, in eastern Williams and McKenzie counties, and in Newporte Field in Renville County (Fig. 10).
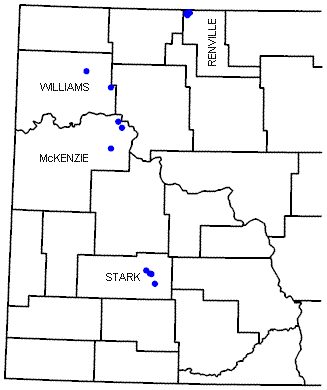
Figure 10. Location of oil and gas wells producing from the Cambrian Deadwood and Ordovician Winnipeg formations.
Tippecanoe Sequence (Ordovician-Silurian)
The Williston Basin contains a well-preserved Ordovician section for the Rocky Mountain region (Foster, 1972). Equivalent strata are thought to have covered a much greater area, once extending at least as far as Nebraska to the south and southeast, but later erosion has removed much of the strata. Deposition was continuous across the Ordovician-Silurian boundary and sedimentation continued at least until Middle Silurian. The top of the sequence is a major erosional unconformity that has removed an unknown amount of strata.
Winnipeg Group
Earliest Tippecanoe Sequence (Fig. 7) sedimentation begins with the Williston Basin connected to the ocean through a southwest trending seaway (Foster, 1972). Sedimentation is recorded by the Black Island, Icebox, and Roughlock formations of the Winnipeg Group. All of these formations were deposited in marginal to shallow marine environments.
The Black Island Formation has two members (Thompson, 1984). The lower member is comprised of two lithofacies, a lower red-bed lithofacies containing quartz arenites and "clayshales", and an upper green quartz wacke. The upper member is also comprised of two lithofacies, a quartz arenite and green quartz wackes. The Icebox Formation, an organic-rich green shale, is thought to be a source rock for Lower Paleozoic reservoirs (Dow, 1974; Williams, 1974). The Roughlock Formation is predominantly a nodular limestone and is transitional with the overlying Red River Formation (LeFever et al, 1987).
The Winnipeg Group is productive on the Nesson anticline and at Richardton and Taylor fields, on the Heart River anticline in eastern Stark County (Figs. 5 & 9). In both areas, production is from Black Island sandstones and natural gas is the dominant hydrocarbon produced.
Bighorn Group
The Big Horn Group conformably overlies the Roughlock Formation of the Winnipeg Group (Fig. 6). The Red River Formation, the basal unit of the group, has been subdivided into two informal members. The lower member is the lower two-thirds of the formation and is composed of fossiliferous and selectively dolomitized limestones. The upper member includes four dolomitized porosity zones; the "D", "C", "B", and "A" zones, in ascending order (Carroll, 1979). The upper three zones are capped by anhydrite beds while the "D" zone is not (Fig. 11). The anhydrites are widespread, as they are present across most of western North Dakota and eastern Montana, and they are present in the center of the basin (Fig. 12). Two depositional models have been proposed to explain the origin of the dolomites and capping anhydritesin the upper Red River Formation. In the first model, the marine waters in the central Williston Basin evaporated to the point that a sabkha developed there (Carroll, 1979) while in the second model, all deposition occurred in subtidal environments (Kendall, 1976). The data can be interpreted to fit either model, hence the controversy.
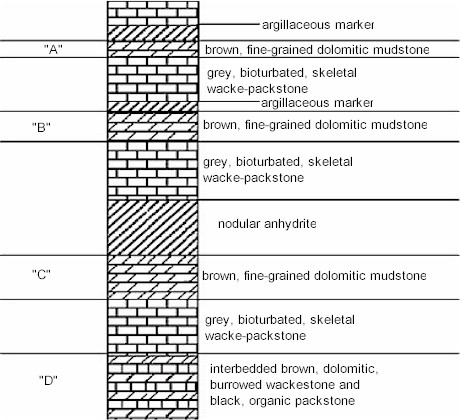
Figure 11. Stratigraphy of the upper Red River Formation The letters correspond to informally named porosity zones. (Modified from Carroll, 1979).
Figure 12. Distribution of the Red River "A", "B", and "C" anhydrites (Modified from Foster, 1972).
In the sabkha model, the "D" zone strata were deposited in a subtidal to intertidal environment and the basal strata of the "C", "B", and "A" zones were deposited in a subtidal environment. The upper strata of the "C", "B", and "A" zones were deposited in a broad supratidal environment, or sabkha (Carroll, 1979). In this model, a series of marine transgressions deposited subtidal to intertidal sediments during the basal phase of each cycle, were followed by a marine regression. In the cases of the "C", "B", and "A" zones, the regression dried out the basin. During "D" zone sedimentation, fresh water infiltrated into the sediments on the tidal flats and mixed with normal marine waters changing the Mg/Ca ratio of the mixed waters enough to dolomitize the sediments. Dolomitization in the upper three zones was different - penecontemporaneous with deposition. The anhydrites were deposited in the high-evaporation environment of the sabkha.
The sabkha model requires that sabkha progradation continued until the center of the basin was completely filled. In the second model of upper Red River sedimentation, deposition occurred in a subtidal environment. In this model, the dolomites and anhydrites were deposited during periods of basin restriction and increasing water salinity (Kendall, 1976). The anhydrites were precipitated from the seawater when a high enough water salinity was reached before conditions returned to a more normal marine environment. Dolomitization would occur because the Mg/Ca ratio of the water increased with the precipitation of the anhydrite and then dolomitization could occur. Another possibility is that the dolomites formed as a result of a later diagenetic event.
The Red River Formation is productive across most of western North Dakota. Most Red River Formation production has been found west of the Nesson anticline, in the deepest parts of the basin, and is associated with structural closures. However, the best porosity is not always coincident with the structure's crest (Longman et al, 1983). The Red River Formation is the second most important hydrocarbon producing horizon in North Dakota and produces hydrocarbons in many fields (Fig. 13). The Stony Mountain Formation conformably overlies the Red River Formation and is comprised of interbedded calcareous shales and argillaceous limestones. The Stony Mountain Formation is rarely productive, but where it is productive it is always associated with a Red River Formation structure. Production usually comes from the Gunton Member which can have a well developed dolomite porosity. The Stonewall Formation is the uppermost formation in the Big Horn Group and conformably overlies the Stony Mountain Formation (Fig. 6). Continuous sedimentation occurred across the Ordovician-Silurian boundary and dolomites and limestones, with thin anhydrite beds near the basin center, were deposited. The Stonewall Formation produces oil and gas from several zones, usually associated with a Red River structure (Fig. 14).
Interlake Formation
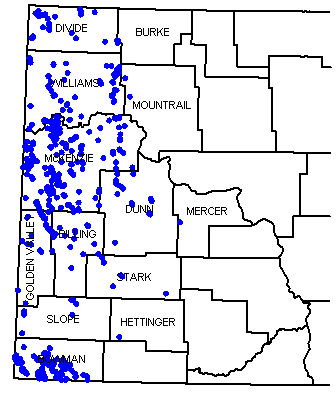
Figure 13. Partial distribution of oil and gas wells producing from the Ordovician Red River Formation.
Figure 14. Partial distribution of oil and gas wells producing from the Silurian Stonewall Formation.
Figure 15. Partial distribution of oil and gas wells producing from the Silurian Interlake Formation.
The Interlake Formation conformably overlies the Stonewall Formation (Fig. 6) and records latest Tippecanoe Sequence deposition. Interlake lithologies are dominated by dolomitic mudstones and dolomites. The formation was exposed from Late Silurian through Early Devonian when karst topography was formed. Various interpretations have been made of Interlake stratigraphy. LoBue (1983) informally subdivided the Interlake Formation into three members, and interpreted the formation as a sequence of sublittoral to supralittoral environments. LoBue also recognized paleosols, and interpreted them as periods of prolonged subaerial exposure. Megathan (Megathan 1987) assigned group status to the Interlake and defined eight formations within it. Megathan interpreted Interlake Group deposition as occurring in a succession of hypersaline (lower Interlake) to freshwater (upper Interlake) environments. In contrast, Inden et al (Inden et al 1988) considered the Interlake to be a formation and interpreted it as a series of low-energy, shallowing-upward cycles deposited in a restricted-marine environment.
The upper Interlake Formation is productive along large structures (Fig. 15), but the controls on production are not well understood. Salt-plugged porosity degrades reservoir performance in some places whereas fracturing has enhanced performance in other areas. The middle Interlake Formation is productive in two fields in Stark County and the lower Interlake Formation produces from two porosity zones, informally named the Salsbury and the Putnam. These two porosity zones produce on major structures in North Dakota and production can be significant, like that at Stoneview Field on the Nesson anticline. Typically, oil with a significant volume of gas is produced from Interlake reservoirs.
Kaskaskia Sequence (Devonian-Mississippian)
Kaskaskia Sequence deposition in the Williston Basin occurred during two transgressive cycles, so the sequence is divided into two parts. Limestones dominate the Kaskaskia Sequence rock-record, but two major evaporate sections are preserved. Rocks of the lower cycle record a northwest connection into the Elk Point Basin while deposition in the upper cycle records a westward connection into the Central Montana Trough.
Lower Kaskaskia Sequence
Elk Point Group
The initial Kaskaskia Sequence transgression into the basin was from the northwest out of the Elk Point Basin (Fig. 16). At the base of the sequence is the Ashern Formation. Lobdell (1984) divided the formation into two members, a lower red dolostone and an upper gray dolostone. The lower member was deposited in a restricted marine environment, whereas the upper member records a change to a less restricted environment as marine transgression continued. Both nodular and bedded anhydrite are present throughout the Ashern, but are more common in the lower member. The Ashern Formation is non-productive in North Dakota. The Winnipegosis Formation conformably overlies the Ashern Formation (Fig. 6) and is dominantly a limestone. In northwestern North Dakota, initial Winnipegosis deposition occurred in the southeastern extent of the Elk Point Basin and on a broad shelf ramp (Fig. 16).
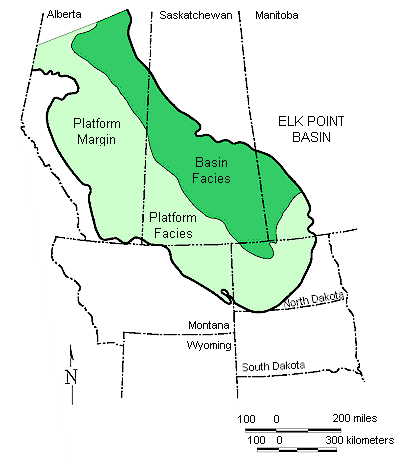
Figure 16. Extent of the Winnipegosis platform and basin in the Elk Point Basin (Modified from Fuzesy, 1982 and Ehrets and Kissling, 1987).
Figure 17. Partial distribution of oil and gas wells producing from the Devonian Winnipegosis Formation.
Later, the shelf differentiated into a basin surrounded by a platform (Ehrets and Kissling, 1987). Shallow water mounds grew on the shelf but, when the shelf differentiated into a basin and platform, the mound biota struggled to keep up with increasing water depths in the rapidly subsiding basin. Eventually, some of the mounds grew into pinnacle reefs.
Some pinnacle reefs produce commercial quantities of oil in the Canadian portion of the Williston Basin, but none do in North Dakota. Commercial Winnipegosis production has been established in North Dakota along the platform margin, at Temple and Hamlet fields, and on the platform, in Round Prairie Field (Fig. 17). Platform margin fields produce from argillaceous carbonates deposited in basin-slope facies; platform fields produce from patch reefs (Ehrets and Kissling, 1987). The Winnipegosis Formation produces oil with a large volume of natural gas.
During latest Winnipegosis deposition, the basin became restricted and the evaporites of the Prairie Formation were deposited. With time, salt deposition spread from the basin onto the basin margins. The restriction continued until eventually, the pinnacle reefs became encased in salt (Kerr, 1988). Dissolution of the Prairie salt is an important local trapping mechanism in the Williston Basin. Beds draped across dissolution edges enhanced closure, as exhibited by Glenburn, Sherwood, and Wiley fields, while two-stage salt dissolution formed the "Nisku Reefs" of northeastern Montana.
Manitoba Group
When the northern seaway into North Dakota reopened and normal marine circulation was reestablished, the Dawson Bay Formation was deposited on a stable, low-relief shelf. In northwestern North Dakota, stromatoporoid-dominated patch reefs formed on an open platform (Dean, 1982). Anhydrite beds in the upper Dawson Bay Formation record renewed restriction of the seaway into the Williston Basin.
The Dawson Bay Formation has produced oil in three North Dakota fields (Fig. 18). At Dolphin and Temple fields, both significant oil fields, porous carbonates pinch out updip on a structural nose (Dean, 1982; Heck, 1987). At Marmon Field, the trap is essentially the same as at Dolphin and Temple fields, but the volume of oil produced is insignificant. In western North Dakota, porosity in the Dawson Bay Formation is generally salt-plugged. However, the localized dissolution of the pore-filling salt can form or enhance a stratigraphic trap as is the case in Dolphin Field. Dawson Bay production is primarily oil, but with a significant volume of associated gas. The Souris River Formation (Fig. 6) conformably overlies the Dawson Bay Formation, and is lithologically similar to it. The formation's interbedded carbonates and evaporites are evidence that the marine restriction, begun during latest Dawson Bay deposition, continued into Souris River deposition.
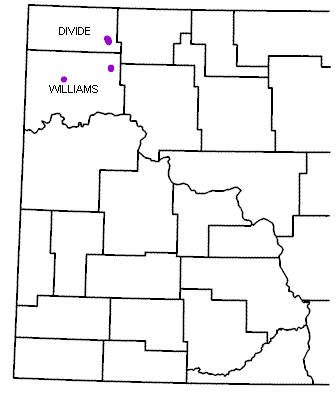
Figure 18. Partial distribution of oil and gas wells producing from the Devonian Dawson Bay Formation.
The Souris River Formation is not considered to be an important oil producing zone because oil has been produced from only one well in Dolphin Field. However, an unknown amount of Souris River Formation production along the Nesson anticline was pooled with that from the overlying Duperow Formation (Pilatzke et al., 1987).
Jefferson Group
The Duperow Formation conformably overlies the Souris River Formation (Fig. 6). Two hypotheses have been proposed to explain the changes in lithology from a lower limestone to dolomite to an upper anhydrite observed in the Duperow Formation. The first model is a series of shallowing-upward sequences where the depositional environment changed from a lower subtidal environment to a middle, restricted environment and ultimately, to an upper intertidal to supratidal environment (Wilson, 1967; Pilatzke et al., 1987). Lithologies similarly changed from burrowed bioclastic limestones containing stromatoporoid banks, to lime mudstones containing ostracods and calcispheres, to bedded anhydrites and silty dolomites.The second model invokes a series of marine restrictions during which salinity increased. Each restriction began in normal to near normal marine environments and culminated in a hypersaline environment in which bedded anhydrites and dolomites were deposited (Ehrets and Kissling, 1985). This model has sometimes been called a brining-upwards sequence.
The Duperow Formation produces from stratigraphic traps in the central Williston Basin, from structural traps along the Nesson anticline, and from combination traps on the Billings anticline. The Duperow Formation also produces on the eastern flank of the Cedar Creek anticline, where truncated porous carbonates are capped by Englewood Formation equivalents. The Duperow Formation is the third largest oil producing zone in the state, after the Madison Group and the Red River Formation, and produces a combination of oil and natural gas (Fig. 19).
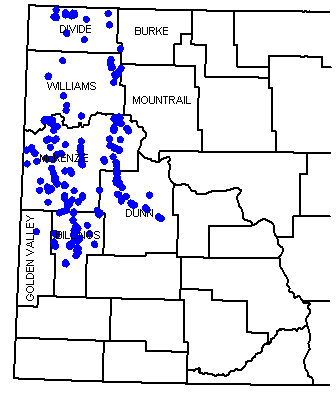
Figure 19. Partial distribution of oil and gas wells producing from the Devonian Duperow Formation.
The Birdbear (Nisku) Formation conformably overlies the Duperow Formation. Loeffler (1982) described the Birdbear Formation as fossiliferous limestones and dolomitic muddy limestones deposited in shallow, normal marine environments, that changed upwards into anhydrites and dolomites deposited in a sabkha environment.
The Birdbear Formation produces oil and natural gas from a variety of traps. Stratigraphic traps include stromatoporoid banks, amphiporid back-bank facies, and locally dolomitized porosities. The formation also produces from small structures on the Nesson anticline and elsewhere (Fig. 20), truncated porosity zones along the east flank of the Cedar Creek anticline, and from two-stage salt dissolution structures in northeastern Montana.
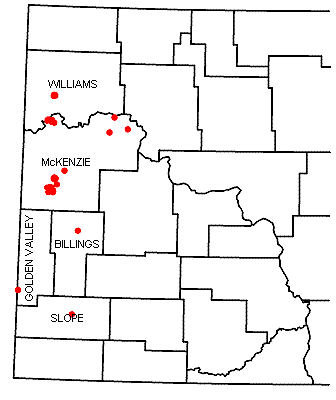
Figure 20. Partial distribution of oil and gas wells producing from the Devonian Birdbear Formation.
Three Forks Formation
The Three Forks Formation conformably overlies the Birdbear Formation (Fig. 6) and was deposited in shallow marine to supratidal environments in a shallow epeiric sea (Dumonceaux, 1984). The Three Forks Formation is primarily a micrite to dolomicrite interbedded with anhydrite. An informal unit, called the Sanish sandstone, can be developed at the top of the Three Forks Formation. The Sanish sandstone produces oil and gas in Antelope Field and is one of the largest oil pools in the state.
Bakken Formation
The Bakken Formation conformably overlies the Three Forks Formation in the basin center, and unconformably overlies it elsewhere (Webster, 1984). Gerhard et al. (Gerhard et al. 1982) thought the Bakken Formation recorded the initial depositional phase of the upper Kaskaskia Sequence. However, Hester and Schmoker (Hester and Schmoker 1985) showed that the Bakken Formation depositional pattern was coincident with the Elk Point Basin. Therefore, the Bakken Formation is included in the lower Kaskaskia Sequence.
The Bakken Formation has three informal members, an upper and a lower black, organic-rich shale, separated by an arenaceous limestone to siltstone. Depositional environments interpreted for the Bakken Formation have ranged from a marine swamp with restricted circulation caused by the prolific growth of organic matter to an offshore marine environment with a stratified water column (LeFever, 1991). The latter environment model is preferred.
The Bakken Formation is an excellent source rock and was considered to be the source rock for most Mississippian reservoirs in the Williston Basin (Dow, 1974; Webster, 1984; Price et al., 1984). Recent work has shown that the Lodgepole Formation was the source of at least some of the Mississippian oils (Osadetz and Snowdon, 1986). Price and LeFever (1994) determined that the Bakken oils were confined to the Bakken source system (uppermost Three Forks Formation to lowermost Lodgepole Formation), and have not migrated into the overlying Mississippian reservoirs as previously thought.
The Bakken Formation is productive on, and near, the Nesson anticline, and along its southwestern depositional limit in Golden Valley and Billings counties (Fig. 21). The upper black shale of the Bakken Formation has poor matrix permeabilities but produces where overpressured and fractured. Production from the middle member is found along the northern Nesson anticline where low porosity and permeability sandstones produce some oil.
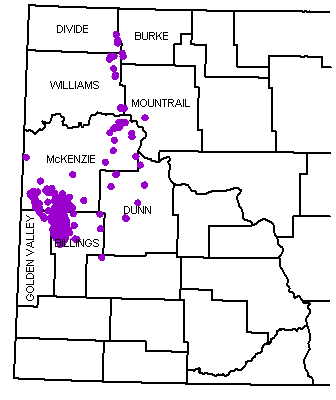
Figure 21. Partial distribution of oil and gas wells producing from the Mississippian-Devonian Bakken Formation.
Upper Kaskaskia Sequence
Madison Group
Deposition of the upper Kaskaskia Sequence began sometime after middle Lodgepole deposition (Fig. 22). At that time, the sedimentation in the basin records a change in sediment source from the Elk Point Basin to the Central Montana Trough (LeFever and Anderson, 1984).
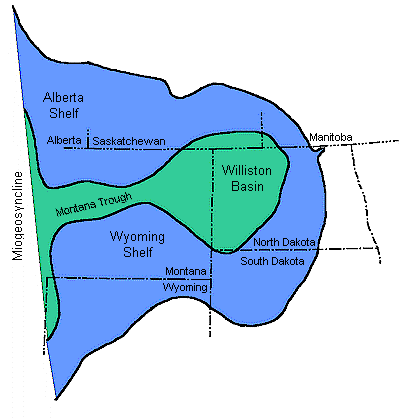
Figure 22. Seaway connection of the Williston Basin to the ocean during Upper Kaskaskia deposition (Modified after Gerhard et al., 1982).
The Madison Group is made up of three formations; the Lodgepole, Mission Canyon, and Charles, in ascending order (Fig. 6). These formations are conformable in the basin center, but exhibit complex intertonguing relationships along the basin margins.
The Madison Group has been divided into a number of informal, wireline log-defined intervals (Harris and others, 1966; Voldseth, 1986). In ascending order, they are the Bottineau, Tilston, Landa, Wayne, Glenburn, Mohall, Sherwood, Bluell, Coteau, Dale, Nesson, Midale, Ratcliffe, and Poplar intervals. Hendricks (Hendricks-1988)subdivided the Ratcliffe interval into several subintervals, the Berentson, Alexander, Flat Lake, Charles C, Lustre, and Eggebrecht (Fig. 23).
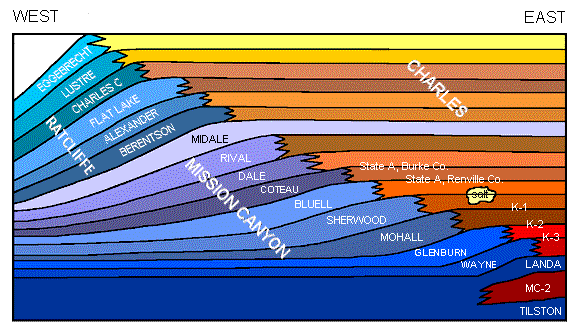
Figure 23. Facies relationships and nomenclature of the Mission Canyon and Charles formations in the Williston Basin (Modified from Hendricks, 1988; Voldseth, 1986; Harris et al., 1966). The blue colors represent carbonate rocks and the reds and yellows represent evaporites.
The Lodgepole Formation (Bottineau interval) conformably overlies the Bakken Formation in the basin center, and unconformably onlaps Upper Devonian strata in both eastern North Dakota and along the Cedar Creek anticline. The formation consists of limestones and dolomites deposited in normal marine to restricted shelf environments (Heck, 1979).
The Lodgepole Formation is a major producing horizon in Manitoba, but significant production in North Dakota was not found until 1994 when the Dickinson Lodgepole pool was discovered. Lodgepole wells in this pool, and several other nearby fields, are capable of producing several thousand barrels per day from Waulsortian bioherms. Bjorlie and Anderson (1978) had postulated a system of lower Lodgepole Waulsortian bioherms in eastern North Dakota deposited in a shallow, normal marine shelf environment, but the existence of Waulsortian bioherms in a basinal environment was completely unknown before the Dickinson Lodgepole discovery. A porosity zone in the middle Lodgepole has produced oil in several Williams County wells, but it was uneconomic (LeFever and Anderson, 1984). In addition to containing good reservoir rock in the Waulsortian bioherms, shale beds and argillaceous limestones in the lower Lodgepole may be an important petroleum source rock (Osadetz and Snowdon, 1986).
The Mission Canyon Formation (Tilston through Dale intervals) consists primarily of limestones interbedded with anhydrites and dolomites. Deposition occurred in environments that ranged from open marine to coastal sabkha and record a regressive sequence (Lindsay, 1988). The Mission Canyon Formation has produced more oil than any other stratigraphic unit in the Williston Basin (Table 1 and Fig. 24).
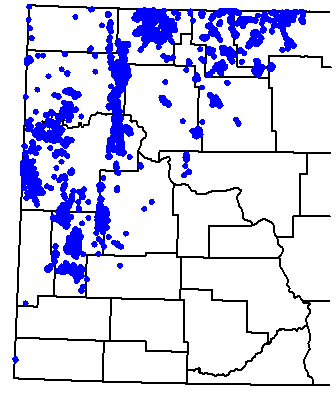
Figure 24. Partial distribution of oil and gas wells producing from the Mississippian Madison Group.
The Charles Formation (Ratcliffe and Poplar intervals) consists of interbedded evaporites and limestones deposited in a restricted marine environment. The Charles Formation records a major marine regression during the upper Kaskaskia Sequence. Lindsay (Lindsay 1988) and Hendricks (Hendricks1988) identified four main types of Mission Canyon traps: 1) combination structural and stratigraphic traps; 2) porous carbonate (usually an island or shoal) pinching out updip into impermeable (intertidal or inter-island) carbonate; 3) porous carbonate facies changing updip into impermeable anhydrite; 4) truncated porous carbonate capped by impermeable Triassic rocks. Approximately 60% of the oil produced in North Dakota has come from the Charles and Mission Canyon formations.
Big Snowy Group
Latest Kaskaskia Sequence deposition is recorded by the Kibbey and Otter formations (Fig. 6). Both formations consist of interbedded sandstones, shales, and limestones. The clastic rocks had an extra-basinal source and mark the influence of the Ancestral Rocky Mountain orogenic event (Gerhard et al., 1982). The Kibbey Formation is productive along the Weldon fault in Montana and from one well in Red Wing Creek Field, North Dakota. In central North Dakota, the unconformity at the top of the Kaskaskia Sequence truncated only the Otter Formation. Elsewhere, variable amounts of Kaskaskia Sequence strata are missing.
Absaroka Sequence
During Absaroka deposition, marine transgressions were from the southwest and deposition was concurrent with tectonic activity southwest of the Williston Basin. Interbedded marginal marine evaporates and terrestrial rocks record sedimentation within the basin.
Minnelusa Group
Deposition of the Tyler Formation (Pennsylvanian) occurred in a slowly subsiding basin and recorded the beginning of the Absaroka Sequence. Sturm (Sturm 1982) divided the Tyler Formation into two informal units, a lower unit of interbedded shales, mudstones, and sandstones; and an upper unit of interbedded limestones, calcareous mudstones, and anhydrites. He interpreted the lower unit as a prograding delta and the upper unit as a barrier island. The latter is capped by rocks deposited in lagoonal and estuarine environments.
The Tyler Formation produces oil from both the barrier island and from channel-fill sandstones and is limited mostly to southwestern North Dakota (Fig. 25) The source rocks for this oil are thought to be the shales within the Tyler (Williams, 1974) but Sturm (Sturm-1982), suggested that some shale and limestone beds should be examined for their source potential.
Spearfish Formation
The youngest oil-producing formation in North Dakota is the Triassic Spearfish Formation (Fig. 6), which unconformably overlies the Madison Group across much of eastern North Dakota. The Spearfish Formation is productive where oil has migrated into it from Madison Group strata.
ZUNI AND TEJAS SEQUENCES
Dakota and Montana Groups
Zuni and Tejas Sequence strata are the youngest rocks to produce hydrocarbons in North Dakota and they produce natural gas (Fig. 6). Zuni Sequence gas production includes small volumes of gas produced from the Newcastle Sandstone (Lower Cretaceous), in LaMoure and Dickey counties, and large volumes of gas from the Eagle and Judith River sandstones (Upper Cretaceous) of the Pierre Formation in Bowman County. These two sandstones produce from thin interbeds of silty sandstone between shales along the Cedar Creek anticline in southwestern North Dakota (Fig. 26). This production was established during the 1920's, making it some of the oldest production in the state (Anderson and Eastwood, 1968).The first hydrocarbons produced in North Dakota came from the youngest strata in the state, glacial drift of the Tejas Sequence. Production was discovered in 1907, near Westhope, and other accumulations were later found near Maxbass and Mohall with enough natural gas being produced to supply the towns of Lansford and Westhope for a time (Bluemle, 1996). However, there is no production from glacial drift today.
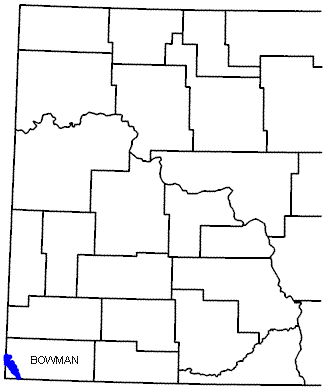
Figure 26. Partial distribution of gas wells producing from the Cretaceous Eagle and Judith River sandstones.
History of Oil and Gas Exploration in North Dakota
Oil and gas exploration in North Dakota has been cyclical with four cycles of exploratory drilling (Fig. 27). The first drilling cycle began in 1951, with the discovery of oil in Williams County. Subsequent drilling defined the Nesson anticline, a 75-mile long structure with nearly continuous production from multiple pay zones. Two major plays, identified in 1953 and 1954, are the Mississippian subcrop play in north-central North Dakota and the Mississippian/Pennsylvanian play in southwestern North Dakota. By the early 1960's, the first exploration cycle had ended and activity focused on development and extension drilling.
The second drilling cycle was in full swing by 1968 following the discovery of shallow Cretaceous oil at Bell Creek Field in the northeastern Powder River Basin. This cycle records the most exploration for Cretaceous targets in North Dakota, and was a response by the industry to the Bell Creek Field discovery.
While the Cretaceous play in North Dakota was not successful, oil was discovered in the Red River Formation in Bowman County and from the Bakken Formation in Billings County during cycle two. While important during cycle two, both discoveries would have a greater influence on cycle three drilling.
The third exploratory cycle began in the mid-1970's and was the most intensive of the three cycles. Hundreds of new fields and pools were discovered during this cycle with much of the drilling concentrated in west-central North Dakota. The intensity of this cycle was the result of many factors. Two of them were the 1972 discovery of Red Wing Creek Field and the 1973 Arab oil embargo.
Red Wing Creek Field is structurally complex, with a pay section greater than 1,000 feet thick. The discovery of this field initiated a major lease play in western North Dakota. Geological and geophysical programs were completed over many of these leases when in 1973, the oil embargo focused the industry on domestic exploration. During the cycle, no new Red Wing Creek fields were found, but the rapid escalation of oil prices made almost any discovery economical. Some of the fields discovered during this cycle are Mondak Field, Little Knife Field, and the Billings anticline complex. These fields produce primarily from Mississippian reservoirs, though all produce from multiple pays, and are the largest oil fields ever discovered in the state. The collapse of oil prices in 1986 brought a rapid and devastating conclusion to cycle three.
Cycle three saw the first Bakken Formation play. Previously, Bakken completions were primarily for salvage, but several Billings County completions in the early 1980's with high initial potentials focused attention on the formation. Development of Bakken reservoirs occurred in Elkhorn Ranch, Buckhorn, and Devil's Pass fields.
Cycle four was initiated by two events, the discovery of the Dickinson Lodgepole Field and the discovery of the horizontally drilled Red River "B" pool in Cedar Hills Field in Bowman County. Cycle four was short-lived as the Lodgepole play has not yet been successfully extended outside the immediate Dickinson area and Cedar Hills Field in Bowman County has been fully developed.
North Dakota is presently in a situation similar to that at the end of cycle three. Drilling remains flat overall, but smaller peaks are seen when a new play is active and lows are seen when there is no active play and crude oil prices are low as they were during mid-1999.
In summary, Cycle one drilling explored mainly for reservoirs along the Nesson anticline; Cycle two activity reflected the northward extension of the Bowman County Red River play and the influence of the discovery of Bell Creek Field; Cycle three drilling was concentrated in the central basin for deep multiple-pay targets and drilling ended when oil prices plummeted during 1986; and Cycle four was a burst of activity centered on two plays, the Lodgepole and horizontal Red River "B" plays. Drilling will probably not increase significantly without a major price increase or a significant new discovery.
Enhanced Oil Recovery
Enhanced Oil Recovery (EOR) encompasses a variety of projects that oil companies employ to increase oil production from a field. These efforts are most often begun when an oil field is near the end of its primary production life. Thus, other names for EOR are secondary recovery or tertiary recovery projects. A typical North Dakota oil field produces only 15-20% of all the oil trapped in the rocks or 15-20% of the original-oil-in-place (OOIP). If conditions are right, an oil company can begin injecting water to displace the trapped oil with a waterflood, or they might drill additional wells in an infill-drilling program to produce some of the 80-85% of the oil that otherwise would be left behind. There are other methods that can be used, but which method will be most effective will depend on the properties of the reservoir. Some of these properties are porosity, permeability, grain size, reservoir pressure, depth, temperature, oil viscosity, and other factors. EOR projects require additional spending by the oil companies. EOR projects can be a good investment because there are fewer risks involved as you already know the oil is there, however there is no guarantee the project will work well enough to get the investment back.
Enhanced oil recovery (EOR) projects in North Dakota have met with varying degrees of success. Some failed to produce any incremental oil while others successfully increased recovery. Most of the unsuccessful EOR projects were attempts to waterflood Madison reservoirs in north-central North Dakota. The failure of these waterfloods is inexplicable because waterfloods in the same strata in Canada have been successful. One explanation is that project operations were conducted differently while another explanation is that reservoir properties in North Dakota differ from similar reservoirs in Canada. Carbonate reservoirs are often inhomogeneous and only a thorough understanding of the reservoir characteristics and careful planning can compensate for these inhomogeneities. The EOR projects attempted in North Dakota are listed in Table II. Each of the listed EOR projects has a unique identifying abbreviation that corresponds to those in figure 28, a location map of all the active units in North Dakota.
In 1983, Chevron Oil Co. attempted to unitize Little Knife Field to institute a CO2 flood for pressure maintenance. A successful pilot study involving five wells had shown that the program would probably be successful (Desch et al., 1984), but the unitization attempt failed because the 80% of the royalty interest owners necessary to ratify a unitization agreement in North Dakota was not attained.
Recent CO2 enhanced recovery programs in Canada have apparently been successful. These successful programs, coupled with the apparent success of the Chevron pilot program at Little Knife Field, might point to a future need for CO2. There are two sources of CO2 presently available to operators in the Williston Basin. The first source is the Wyoming Thrust Belt, where CO2 is produced together with other natural gasses. The second source is the coal gasification project at Beulah, North Dakota where CO2 is a byproduct of the process. A pipeline is being built to transport CO2 to the Weyburn Field in Saskatchewan and startup of a CO2 flood may begin during 1999. The pipeline's route takes it past many other fields that are suitable for CO2 programs and perhaps some other fields will be CO2-flooded soon.
Source: North Dakota Oil & Gas Division
Selected References for North Dakota Petroleum
Brostuen, E.A., 1981, Petroleum - A Primer for North Dakota: North Dakota Geological Survey Educational Series No. 13. 34 p.
Carlson, C.G., Bakken, W.E., and Kume, J., 1960, (Repr. from the Compass, v. 37, no. 2, p. 123-143), Subsurface Geology and Development of Petroleum in North Dakota: North Dakota Geological Survey Bulletin No. 34.
Fischer, D.W., and Rygh, M.E., 1989, A Synoptic Overview of the Bakken Formation in Portions of Billings, Golden Valley, and McKenzie Counties, North Dakota: North Dakota Geological Survey Report of Investigation No. 89. 14 p.
Gaswirth, S.B. and K.R. Marra, 2015, U.S. Geological Survey 2013 assessment of undiscovered resources in the Bakken and Three Forks Formations of the U.S. Williston Basin Province: AAPG Bulletin, v. 99, no. 4, p. 639-660.
Gerhard, L.C., Anderson, S.B., LeFever, J.A., and Carlson, C.G., 1982 (Repr. from Am. Assoc. Petroleum Geologists Bull., v. 66, no. 8, p. 989-1020), Geological Development, Origin, and Energy Mineral Resources of the Williston Basin, North Dakota: North Dakota Geological Survey Miscellaneous Series No. 63.
Gonzalez, M.A., Burke, R.B., and Diehl, P.E., 2003, Black Gold: The Role of the Geological Survey in the Exploration, Discovery, and Development of Oil Prospects and their Economic Implications to North Dakota: North Dakota Geological Survey Educational Series No. 29. PowerPoint presentation.
Laird, W.M., 1951 (Repr. from World Oil, June-July 1951, v. 132, no. 7, p. 73- 75, 84; v. 133, no. 1, p. 83-85), Discovery Heightens Interest in North Dakota Geology: North Dakota Geological Survey Report of Investigation No. 3.
Laird, W.M., and Folsom, C.B., Jr., 1956 (Repr. from World Oil, March and June 1956), North Dakota's Nesson Anticline: North Dakota Geological Survey Report of Investigation No. 22. 12 p.
Laird, W.M., and Towse, D.F., 1949 (Rev. 1951, 1953), Stratigraphy of North Dakota with Reference to Oil Possibilities: North Dakota Geological Survey Report of Investigation No. 2. 2 pl. with text.
LeFever, J.A., 2007, Bakken Middle Member Lithofacies: North Dakota Geological Survey Geologic Investigation No. 45. 6 maps series.
LeFever, J.A., 2008, Structural Contour and Isopach Maps of the Bakken Formation in North Dakota: North Dakota Geological Survey Geologic Investigation No. 59. 5 map series.
LeFever, J.A., 2013, Geotechnical Insights of the Bakken: North Dakota Geological Survey Geologic Investigation No. 170.
LeFever, J.A., LeFever, R.D., & Nordeng, S.H., 2013, Reservoirs of the Bakken Petroleum System: A Core-based Perspective: North Dakota Geological Survey Geologic Investigation No. 171.
Leonard, A.G., 1920, Possibilities of Oil and Gas in North Dakota: North Dakota Geological Survey Bulletin No. 1. 9 p.
Nesheim, T.O., 2020, History of exploration and development in the middle Three Forks (2nd bench): North Dakota Department of Mineral Resources Geo News, vol. 47, no. 2, p. 18-20.
Nesheim, T.O., 2020, Evaluation of Water-Cuts from Middle and Lower Three Forks Production (2nd and 3rd benches): Western North Dakota: North Dakota Geological Survey Geological Investigation No. 245, 18 p.
Nesheim, T.O., 2024, Review of Non-Bakken/Three Forks Oil and Gas Drilling Activity in Western North Dakota Since 2013: Department of Mineral Resources Geo News Newsletter, vol. 51, no. 2, p. 2-4.
Nesheim, T.O., and Starns, E.C., 2024, Middle Three Forks Development in the Bakken-Three Forks Petroleum System, Rate Acceleration or Resource Addition?: North Dakota Geological Survey Report of Investigation No. 135, 24 p.
Nordeng, S.H., 2013, Petroleum Systems in the Williston Basin: North Dakota Department of Mineral Resources Geo News Newsletter, vol. 40, no. 1, p. 8-13.
Nordeng, S.H., LeFever, J.A., Anderson, F.J., Bingle-Davis, M., and Johnson, E.H., 2010, An Examination of the Factors that Impact Oil Production from the Middle Member of the Bakken Formation in Mountrail County, ND: North Dakota Geological Survey Report of Investigation No. 109.
Starns, E.C., and Nesheim, T.O., 2024, Middle Three Forks Development Impact and Resource Potential in Northeastern McKenzie County, North Dakota: North Dakota Geological Survey Report of Investigation No. 136, 42 p.
United States Geological Survey (USGS), 2021, Assessment of Undiscovered Continuous Oil Resources in the Bakken and Three Forks Formations of the Williston Basin Province, North Dakota and Montana: Fact Sheet 2021–3058.
Potash / Salt
There are three main salt deposits within the Williston Basin of North Dakota: halite, potash, and Glauber salt or mirabolite. Halite (sodium chloride or table salt) and potash occur in thick deposits in the deep subsurface in the western part of the basin. On the other hand, Glauber salt occurs at or within 70 feet of the surface throughout North Dakota.
Halite beds are found in the Prairie (Devonian), Charles (Mississippian), Opeche (Permian), Spearfish (Permian-Triassic), and Piper (Jurassic) formations. Potash beds are known to occur in the Prairie Formation (Devonian). Sodium sulfate deposits are known to occur in Holocene lacustrine sediments.
Halite
Halite occurs in as many as 11 beds ranging in age from Devonian to Jurassic, occurring at depths of 3,600 (McHenry County) to 12,600 feet (McKenzie County) in North Dakota. In the past, salt was produced from solution mines at a site near Williston and a site in Bowman County.
Potash
Potash may be mined from North Dakota in beds of sylvite (potassium chloride) or sylvinite (mixtures of potassium chloride and sodium chloride). Approximately 50 billion tons of potash occur in the Prairie Formation (Devonian) in North Dakota. These evaporites were deposited in a trough that extends from the Northwest Territories in Canada to northeastern Montana and northwestern North Dakota. Potash beds occupy an area of 11,000 square miles that extends from the Montana border to central Bottineau County and from the Canadian border to central Dunn County. This salt interval reaches its maximum thickness of over 500 feet in Burke County. The potash portion of the salt section has a gross thickness of 83 feet.
Thick salt deposits accumulated over the wide expanse of the Elk Point Basin during the Middle Devonian some 400 million years ago, when waters extended down to the northwestern portion of modern-day North Dakota. At times, when water circulation was impeded, potassium salts would precipitate out of dense brine and accumulate into distinct layers within the larger salt body. These potash-rich zones are found in the subsurface in areas of Saskatchewan, Manitoba, Montana, and North Dakota in a rock unit named the Prairie (Evaporite) Formation.
The Prairie Formation was deposited in broad horizontal layers, but due to the structural development of the Williston Basin, the Prairie Formation dips inward toward the center of the basin. As a result, the Prairie Formation is closer to the surface in Saskatchewan than it is in North Dakota. Before 2014, it was assumed that the potash layers in North Dakota were extensions of the Esterhazy, Belle Plaine, and Patience Lake Members which are mined in Saskatchewan. At the time, the potash layer referred to as the Mountrail Member in North Dakota had not been correlated across the Canadian border but was considered likely to be the stratigraphic equivalent of the Patience Lake Member (Anderson & Swinehart, 1979). Subsequently, a NDGS investigation of the Prairie Formation made these cross-border correlations and found that the Mountrail member was a distinct layer that does not extend into Saskatchewan and overlies the Patience Lake member. It was also found that the White Bear Member, present in the southeastern corner of Saskatchewan, but thinning to the status of a marker bed in the Canadian mining region (Fuzesy, 1982), is the most prominent of the potash-containing beds in North Dakota. Finally, a sixth, thin potash bed of very limited areal extent was identified stratigraphically above the other members and was named the White Lake Member.
The main reason that North Dakota's potash deposits have not been utilized is because these same beds are found at much shallower depths which enable the use of both conventional mining as well as solution mining in southern Saskatchewan. There are currently eleven potash mines in Saskatchewan. Mosiac operates a large potash plant that processes brines from a series of solution wells near Belle Plain, Saskatchewan. It is the oldest and largest potash solution mine in the world. Potash is primarily used in the manufacture of fertilizer although testing is currently being done to determine the feasibility of a potash-based water-softening salt.
A typical potash solution mine involves a process of injecting fresh water and recovering brine from a set of two vertical wells to form caverns in the salt below a potash deposit. The caverns are enlarged until open communication between the two wells exists and sumps are created below the wells where insoluble impurities can be held. Once the wells are connected, hot brine is injected into one well where it dissolves potash from the roof and walls before it is extracted through the other well. The process works its way up through the potash layer with an injected oil blanket used to prevent uncontrolled vertical migration. Back at the surface, the potash-containing brine is recrystallized in ponds by natural or mechanical cooling, with both potash and salt (primarily NaCl) being brought to the surface.
Sodium Sulfate (Glauber Salt)
Many of the lakes in northwestern North Dakota contain brine, water with a high salt content, throughout much of the year. Sulfate concentrations exceed 100,000 milligrams per liter (mg/l) in many of these lakes. The salt content of these lakes typically is lowest in the spring and increases throughout the year until it reaches a point in the late summer and fall when evaporation and cooling water temperatures cause Glauber salt to be precipitated. Many of these are playa lakes; that is they routinely go completely dry in the late summer and fall. It is common for 2 to 8 inches of Glauber salt to form on these lake bottoms in the fall. Due to the high salinity of the water, most of these lakes contain little or no aquatic life, except for brine shrimp.
Scientists believe that sodium sulfate crystals (in the form of mirabolite) began forming in some of the shallow lake bottoms in the northwest portion of the state shortly after the end of glaciation approximately 12,000 years ago. Many of the lakes in this area contain layers of sodium sulfate crystals that are from a few inches to over 20 feet thick. The thickness of lacustrine sediments beneath these lakes is quite variable from lake to lake and, at least in a few cases, quite variable within a given lake. Two lakes (Grenora #2 and Miller Lake) contain an average of 15 to 20 feet of lacustrine sediments (including mud and salt) and also have depressions that contain 50 to 70 feet of clay and salt. These depressions are thought to have been created when large iceblocks trapped in the glacial sediment slowly melted creating voids. As a result of the variability of thickness and of the thickness and extent of Glauber salt layers, numerous holes must be drilled at each lake to accurately determine the amount of salt in a given deposit. Information obtained by a 1934 Federal Emergency Relief Administration project, a 1952 U.S. Bureau of Mines study, and a North Dakota Geological Survey project were used to determine that there is an estimated 46 million tons of Glauber salt beneath fifteen lakes in northwestern North Dakota.
Historically, the main use of Glauber salt has been in the production of Kraft paper. However, in recent years the detergent industry has become the main consumer of this salt in its use as a filler for powdered laundry detergent. A Canadian company recently began converting sodium sulfate to sodium bicarbonate by adding anhydrous ammonia and carbon dioxide to the salt. A byproduct of this procedure is ammonium sulfate, a marketable fertilizer.
Selected References for North Dakota Salt
Halite
Anderson, S.B., and Hansen, D.E., 1957, Halite deposits in North Dakota: North Dakota Geological Survey Report of Investigation No. 28, 3 pl. RI-28
Burke, R.B., 2001, Some Aspects of Salt Dissolution in the Williston Basin of North Dakota: NDGS Newsletter, vol. 28, no. 1, p. 1-5.
Fischer, D.W., and Anderson, S.B., 1984, Little-known mid-Paleozoic salts of northwestern North Dakota: North Dakota Geological Survey Report of Investigation No. 83, 18 p. RI-83
Potash
Anderson, S.B., and Swinehart, R.P., 1979, Potash salts in the Williston Basin, U.S.A.: North Dakota Geological Survey Report of Investigation No. 68, 18 p. RI-68
Carlson, C.G., and Anderson, S.B., 1966, Potash in North Dakota: North Dakota Geological Survey Miscellaneous Series No. 26, 12 p. MS-26
Kruger, N.W., 2014, The Potash Members of the Prairie Formation in North Dakota: North Dakota Geological Survey Report of Investigation No. 113. RI-113
Kruger, N.W., 2016, Potash Mineralogy Estimates Based On Quantitative Log Evaluation of the Prairie Formation in North Dakota: North Dakota Geological Survey Report of Investigation No. 116. RI-116
Kruger, N.W., 2018, K2O Grades of the Potash-containing Members of the Prairie Formation, Crosby 100K Sheet, North Dakota: North Dakota Geological Survey Geologic Investigation No. 209, 6 map series. GI-209
Kruger, N.W., 2019, K2O Grades of the Potash-containing Members of the Prairie Formation, Kenmare 100K Sheet, North Dakota: North Dakota Geological Survey Geologic Investigation No. 215, 6 map series. GI-215
Kruger, N.W., 2019, Prairie Formation Salt Isopach and Depth Maps, North Dakota: North Dakota Geological Survey Geologic Investigation No. 221, 3 map series. GI-221
Kruger, N.W., 2020, K2O Grades of the Potash-containing Members of the Prairie Formation, Mohall 100K Sheet, North Dakota: North Dakota Geological Survey Geologic Investigation No. 247, 4 map series. GI-247
Kruger, N.W., 2021, K2O Grades of the Potash-containing Members of the Prairie Formation, Minot 100K Sheet, North Dakota: North Dakota Geological Survey Geologic Investigation No. 258, 3 map series. GI-258
Kruger, N.W., 2023, K2O Grades of the Potash-containing Members of the Prairie Formation, Stanley 100K Sheet, North Dakota: North Dakota Geological Survey Geologic Investigation No. 268, 5 map series. GI-268
Kruger, N.W., 2023, K2O Grades of the Potash-containing Members of the Prairie Formation, Garrison 100K Sheet, North Dakota: North Dakota Geological Survey Geologic Investigation No. 274, 2 map series. GI-274
Kruger, N.W., 2024, K2O Grades of the Potash-containing Members of the Prairie Formation, Parshall 100K Sheet, North Dakota: North Dakota Geological Survey Geologic Investigation No. 274, 2 map series. GI-280
Kruger, N.W., 2025, A 10,000 Foot* Overview of What's New(s) in Potash: Department of Mineral Resources Geo News newsletter, vol. 52, no. 1, p. 18-19.
Kruger, N.W., 2025, K2O Grades of the Potash-containing Members of the Prairie Formation, Williston 100K Sheet, North Dakota: North Dakota Geological Survey Geologic Investigation No. 283, 6 map series. GI-283
Kruger, N.W., 2025, K2O Grades of the Potash-containing Members of the Prairie Formation, Watford City 100K Sheet, North Dakota: North Dakota Geological Survey Geologic Investigation No. 286, 5 map series. GI-286
Glauber Salt
Burr, A.C., McMillan, W.W., and Budge, C.E., 1951, Sodium sulfate, North Dakota resources, nature-development-utilization: North Dakota Research Foundation, 115 p.
Grossman, I.G., 1949, The sodium sulfate deposits of western North Dakota, a progress report: North Dakota Geological Survey Report of Investigation no. 1, 66 p. RI-1
Lavine, Irvin, Report of Sodium Sulfate Investigation--Report to the Federal Emergency Relief Administration, Project no. S-F2-48: University of North Dakota, 38 p.
Lavine, Irvin, and Feinstein, Herman, 1935, Natural deposits of sodium sulfate in North Dakota: University of North Dakota School of Mines, 8 p.
Murphy, E.C., 1996, The sodium sulfate deposits of northwestern North Dakota: North Dakota Geological Survey Report of Investigation No. 99, 78 p. RI-99
Witkind, I.J., 1952, The localization of sodium sulfate deposits in northeastern Montana and northwestern North Dakota: American Journal of Science, v. 250, p. 667-676.
Rare Earth Elements / Critical Minerals
U.S. coal is currently being evaluated as a potential alternative source of rare earth elements (REE) and other critical minerals. Nonfuel minerals identified as critical by the U.S. Department of the Interior are those that are from a supply chain that is vulnerable to disruption and essential in the manufacturing of a product, the absence of which would have substantial consequences for the U.S. economy and national security. Critical mineral commodities, especially rare earth elements (REE), are increasingly vital components in the manufacturing of modern products, especially those needed for electronics, electrified energy infrastructure components, and advanced defense technologies.
The most recent list (DOI, 2022) includes aluminum, antimony, arsenic, barite, beryllium, bismuth, cesium, chromium, cobalt, fluorspar, gallium, germanium, graphite, hafnium, indium, iridium, lithium, magnesium, manganese, nickel, niobium, palladium, platinum, rhodium, rubidium, ruthenium, tantalum, tellurium, tin, titanium, tungsten, vanadium, zinc, zirconium and the sixteen rare earth elements (REE). The REE (lanthanum, cerium, praseodymium, neodymium, samarium, europium, gadolinium, terbium, dysprosium, holmium, erbium, thulium, ytterbium, lutetium, scandium, and yttrium) are often discussed as a group because of their similar chemical properties and the fact they typically occur together in nature.
The U.S. Department of Energy has made significant investments in evaluating whether extraction from coal could supply the country with some of these critical mineral commodities, especially the REE. Lignite, the lowest rank of coal, has the advantageous characteristic of easily uptaking some critical minerals from fluids moving through the rock column.
The North Dakota Geological Survey (NDGS) began a study of rare earth element concentrations in North Dakota lignites in 2015. Since the beginning of the NDGS critical mineral project, the primary goal has been to identify the stratigraphic position of elevated critical mineral concentrations, determine the methods by which the critical minerals were concentrated, and generate an exploration model that can be utilized by the mineral industry. The Survey identified significant enrichment of REE in lignites within or just below the lower Bear Den Member of the Golden Valley Formation. REE and other critical minerals were likely present in low to moderate abundances in the siliciclastic sediments of the Bear Den Member, but downward-percolating acidic waters mobilized ions from primary and secondary mineral grains and leached them from the upper part of the profile and redistributed them into its lower portions, concentrating them where lignites occur and preferentially incorporate them into organic complexes. This interval is identifiable in the field as a thick kaolinitic zone recording an ancient weathering profile created during an unusually warm climate event called the Paleocene-Eocene thermal maximum. The Rhame bed at the top of the Slope Formation is another well-studied paleosol, with a similar thick zone of kaolinite with a bed of silcrete, and also represents an ancient weathering profile. The Rhame bed may have experienced a similar degree of weathering as to that of the Bear Den Member and is proximal to the highly enriched coals below the Harmon/Hanson coal beds.
NDGS Rare Earth Elements & Other Critical Minerals reports and articles
Sand & Gravel
Since the earliest days of the North Dakota Geological Survey (NDGS), sand and gravel has been one of the important mineral resources that we have evaluated.
The NDGS first reported on the production of the state’s mineral resources in the Mineral Statistics portion of the Second Biennial Report of the State Geological Survey of North Dakota, published in 1902 by North Dakota’s second State Geologist, Frank A. Wilder. In this report, an accounting of the production of $5,000 of stone and an undetermined amount of gravel was noted. Wilder commented later in the report on North Dakota’s vast gravel resources and stated: “It is impossible to estimate the value of the great gravel deposits of North Dakota, from which thousands of cars are taken annually for railroad ballast.”
As part of both our county and urban surface geologic mapping programs, we have identified where sand and gravel deposits are located. In doing so, we have noted the various landforms that are most likely to contain economically viable sand and gravel deposits.
Three-fourths of North Dakota is covered by glacial sediments. These glacial deposits contain sand and gravel as either outwash or as isolated lenses of sand and gravel within till. Beach ridges and deltas that formed along glacial lakes Agassiz and Souris are also important sources of sand and gravel. Pliocene to Holocene age sand and gravel deposits also occur as terrace deposits, and less commonly as pediments, in the western part of the state. Sand and gravel is the third largest mineral industry after oil and gas and lignite.
Grain size and the quality of sand and gravel are limiting factors that determine whether or not a sand and gravel deposit is developed. The quality of the gravel can be highly variable within a given deposit. The various uses of sand and gravel, such as fill drainage, well screen, concrete, etc., have variable tolerances for the quality of sand and gravel. Usually, the percentage of shale in sand and gravel is the limiting factor for its usefulness. This is especially true for concrete where shale pebbles tend to "pop out" or be "plucked" by the actions of freeze-thaw. In addition, both volcanic and carbonate pebbles may chemically react with the pavement causing zones of weakness, etc. Transportation of sand and gravel is expensive and often makes up the major share of the cost per ton. In areas where good quality sand and gravel is not available, it may be more economical, for some purposes, to crush rocks (sandstones) in the area.
As a commodity, sand and gravel is cheap. The average free-on-board plant price in 2017 was about $8.60 per ton (U.S. Geological Survey, 2018). Transportation costs add significantly to this figure, especially over distances of 20 miles or more, so it makes good economic sense to have the sand and gravel source as close as possible to its point of use. This is why NDGS geologists have been eyeing local sand resources as potential alternatives to the much pricier out-of-state proppant sands that are a vital component of the hydraulic fracturing of oil and gas wells in the Williston Basin (Anderson, 2011, 2018).
Thanks to North Dakota’s glacial past, abundant sand and gravel deposits can be found across the entire state. To meet the needs of current and future industries in North Dakota, responsible stewardship of these resources will be required, as these resources continue to be explored and developed.
Selected References for North Dakota Sand and Gravel
Anderson, F.J., 2010, Locations of Sand and Gravel Sites in North Dakota: North Dakota Geological Survey Geologic Investigation No. 130, 1:500,000 scale map. GI-130
Anderson, F.J., 2011, Dunes on the Wind-Swept Prairie: North Dakota’s Eolian Sands: Department of Mineral Resources Geo News Newsletter, vol. 38, no. 2, p. 3-7.
Anderson, F.J., 2012, Sand and Gravel Resources Show Record Production in North Dakota: Department of Mineral Resources Geo News Newsletter, vol. 39, no. 2, p. 17-22.
Anderson, F.J., 2018, Eolian sands in North Dakota evaluated for use as natural sand proppant for oil & gas wells: North Dakota Geological Survey Geologic Investigation 207, 70 p. GI-207
Anderson, F.J., 2021, Deltas and Dunes: The Sand and Gravel Legacy of Glacial Lake Souris in North-Central North Dakota: Department of Mineral Resources Geo News Newsletter, vol. 48, no. 1, p. 22-23.
Arndt, B.M., 1977, Stratigraphy of offshore sediment – Lake Agassiz, North Dakota: North Dakota Geological Survey Report of Investigation 60, 58 p., 3 pls. RI-60
Maike, C.A., 2017, Surface Mapping of Sand & Gravel and LiDAR Applications: North Dakota Department of Mineral Resources Geo News Newsletter, vol. 44, no. 1, p. 18-19.
Manz, L.A., 2019, Economic Value of Glacial Stratigraphy: North Dakota Department of Mineral Resources Geo News Newsletter, vol. 46, no. 1, p. 23-27.
Moran, S.R., 1973, Sand and gravel; in Mineral and water resources of North Dakota: North Dakota Geological Survey Bulletin 63, p. 119-122.
North Dakota County Bulletins No. 36-80, Parts 1-3: North Dakota Geological Survey.
U.S. Geological Survey, Crushed Stone Statistics and Information, Crushed Stone Statistics and Information | U.S. Geological Survey (usgs.gov)
Shallow Gas
Shallow natural gas occurs within several near-surface geologic environments in North Dakota. Occurrence has been observed or inferred to originate within glacial sediments accumulating as the result of glaciotectonic processes and anthropogenic means. Geologic conditions and potential sourcing consist of a subcrop of Cretaceous marine sediments scoured and mantled by glacial sediments with contained organics as a potential source. Glaciotectonic ice-thrust masses, several square kilometers in areal extent, and structures within Cretaceous units may impose structural control on shallow subsurface fluid flow. The advance and retreat of glacial ice across the northern Great Plains and subsequent sediment unloading may influence the development of horizontal partings and vertical fracturing within glacial sediments and underlying sedimentary strata providing conduits for shallow gas flow. Gas-rich deposits of sodium sulfate up to 80 feet thick beneath 15 playa lakes in northwestern North Dakota consist primarily of black, organic-rich clays and crystalline layers of sodium sulfate of Holocene age. Borehole gas emanations and gas pockets beneath salt layers were observed along with gas escaping from vents on the bottom of Miller Lake in Divide County. Anthropogenic methane generation within landfills occurs in Minot, Harvey, Williston, Grand Forks, and Hillsboro.
Natural Gas – A mixture of hydrocarbons and varying quantities of nonhydrocarbons that exists either in the gaseous phase or in solution with crude oil in natural underground reservoirs. Natural gas is most commonly odorless, but may be associated with hydrogen sulfide (H2S) in the natural environment or methyl mercaptan (CH3SH), which is artificially added to commercial natural gas, to provide an easily recognizable odor.
Shallow Gas – Natural gas that is produced from shallow gas reservoirs and sediments typically of Cretaceous age and younger in North Dakota that range in depth from zero to 5,000 feet.
Methane – The primary constituent of natural gas; colorless and odorless, chemically composed of one carbon and four hydrogen atoms (CH4) that forms from either: the earth’s mantle, the thermal maturation of organic matter, or the bacterial degradation of organic matter in the shallow subsurface.
The North Dakota Geological Survey has received several stories in the form of short anecdotes relating to shallow gas occurrences across the state. After the publication of a shallow gas article by the Associated Press (May 30, 2007) and related media coverage, the NDGS has received over 60 individual anecdotal reports from private residential well owners. These reports relate to natural gas occurrences within private water supply wells.
Stories of shallow natural gas occurrences have come from nearly all parts of North Dakota. Several have suggested a relationship between gas occurrences and shallow coals. A worker from the Dickinson area reported:
“I know that one of our water wells that the plant had drilled in the 1960s produced methane gas. It [the gas] was fed into a large water tank and fed into the plant. As I worked in the office, at times when the right person would come along, I would ask them if they thought water would burn and invariably they would answer no. To their great surprise, I would open the faucet, strike a match, and behold the water would burn! Of course, it was methane from the coal vein it [the water] was coming from. I used to have a lot of fun with that over the years.”
Many of the accounts are quite colorful such as the story provided by a family in Burke County:
“While visiting our parents in the 1950s in Bowbells, North Dakota, we experienced the event of shallow gas. Each morning my dad would gather whichever grandchild was visiting to show them the fire in the bathroom sink. No one was to run any water until everything was set. Then dad would light a match, turn on the faucet, and presto, there would be fire! All of the grandchildren were treated to this magic when they visited Bowbells and still talk of it when they get together.”
Several stories of shallow natural gas occurrences have been reported from the Bottineau and Renville County areas, where several historical accounts have been documented. One rural Bottineau County resident wrote:
“I grew up on a farm in Bottineau County where we could light a match to our water and it would flame. At times it would pop, sputter, and the force would be so great it would knock a cup out of your hand. Whenever someone would visit, my dad would show them how our water would flame.”
Another rural Renville County resident recently wrote:
“When I was a teenager back in the 1950s, I remember my dad lighting the bottom of the pump jack on his deep well and it would burn for some time, the water would come out somewhat fizzy if I recall correctly.”
There are likely to be hundreds, perhaps thousands, of such occurrences and stories that North Dakotans have experienced over the last century. These stories and related anecdotal information about the occurrence of shallow natural gas can be of great interest to the natural gas explorationist and geologist, by providing additional clues to possible origins and potential areas of accumulation of shallow natural gas that may be located in the state. We are most thankful to the numerous residents who have shared their stories with us and we hope that more will do the same and share their stories with us.
Uranium
Western North Dakota contains several areas of known radioactive mineral deposits. There are at least 21 areas in western North Dakota that contain uranium, primarily within lignites, sandstones, or carbonaceous mudstones.
Uranium exploration began in North Dakota in 1948. Investigations done from the late 1940s to the late 1970s discovered several large areas of increased radioactivity in Bowman, Slope, Stark, Billings, and Golden Valley counties. Uranium and other radioactive elements were often found associated with beds of lignite. These low-grade ore deposits often ranged from 0.005 to 0.2 percent uranium. It is theorized by many geologists that these radioactive elements were released during the alteration of volcanic glass. These radioactive elements were then leached by groundwater into the underlying rocks until a change in pH and or Eh caused them to precipitate, often in a coal or organic-rich lenses in sandstone. Early on it was noted that radioactive lignites often were overlain by sandstones.
Discovery of uraniferous lignite deposits in western North Dakota by federal scientists led several energy companies to explore for uranium in western North Dakota during the 1950s. Beginning in 1956, a few hundred tons of uraniferous lignite was shipped from North Dakota to processing plants. The mills were set up to process uraniferous sandstones and had difficulty processing the low-grade ore lignites. Beginning in 1962, this problem was rectified by burning the uraniferous lignite in pits at the mine site, often by burning the bed in place after the overburden had been removed. The process reportedly took from 30 to 60 days and diesel fuel and old tires were often mixed with the lignite to assure that it would burn sufficiently. The ash from the mines was then sent to Belfield or Griffith where it was further reduced by burning in kilns. The resulting ash was then shipped to mills in South Dakota, Colorado, and New Mexico. Uranium mining took place in southwestern North Dakota from 1962 to 1967 (Karsmizki, 1990). At least seven, and possibly as many as fourteen uraniferous lignite mines operated in the state in the 1960s. We will likely never know the exact number of mines because records were poorly kept for several reasons including national security, and most were relatively small, short-lived operations. Typically, these mines were shallow pits excavated to the top of uraniferous (uranium-bearing) lignites. Mining was discontinued in 1967 after a total production of approximately 85,000 tons of ore resulting in 270 tons of "yellow cake" (U3O8).
Renewed interest in uranium in the mid-1970s resulted in several uranium investigations centered in the Chalky Buttes (Slope County) and Gascoyne (Bowman County) areas. In 1976, mineral companies renewed uranium exploration activities in western North Dakota when uranium prices reached $40 per pound. Between 1976 and 1980, the ND Geological Survey issued 22 uranium exploration permits and companies drilled almost 1,400 exploration holes. Most of these holes were drilled in Slope, Bowman, Adams, Billings, and Stark counties.
The accident at the Three Mile Island nuclear plant in Pennsylvania in March of 1979 effectively halted all uranium exploration in the United States. Orders for new power plants ceased and most uranium exploration in the region came to a standstill as many energy companies disbanded their mineral divisions.
While any future uranium mining in North Dakota is speculative, what is clear is both the federal government and the State of North Dakota have much more stringent environmental laws than were in effect five decades ago. These laws would protect the health and safety of the miners as well as local landowners.
Selected References for North Dakota Uranium
Beroni, E.P. and Bauer, H.L., Jr., 1952, Reconnaissance for uraniferous lignites in North Dakota, South Dakota, Montana, and Wyoming: United States Atomic Energy Commission, Technical Information Service Extension, TEI-123, 93 p.
Denson, N.M., Bachman, G.O., and Zeller, H.D., 1959, Uranium-bearing lignite in northwestern South Dakota and adjacent states: United States Geological Survey Bulletin 1055-B, p. 11-57.
Denson, N.M. and Gill, J.R., 1965, Uranium-bearing lignite and carbonaceous shale in the southwestern part of the Williston Basin - a regional study: United States Geological Survey Professional Paper 463, 75 p.
Karsmizki, K.W., 1990, U3O8, Uranium industry context statement: prepared for UNDAR-West by Western History Research, Bozeman, Montana, 79 p.
Moore, G.W., Melin, R.E., and Kepferle, R.C., 1959, Uranium-bearing lignite in southwestern North Dakota: United States Geological Survey Bulletin 1055-E, p. 147-166.
Murphy, E.C., 2007, Uranium Deposits in Southwestern North Dakota: North Dakota Geological Survey Geologic Investigation No. 40. GI-40
Murphy, E.C., 2007, After an Absence of Nearly 30 Years, Interest in North Dakota's Uranium Deposits is Back: Department of Mineral Resources Geo New Newsletter, vol. 34, no. 2, p. 1-4.
Murphy, E.C., 2008, Uranium Exploration Permits Issued: Department of Mineral Resources Geo News Newsletter, vol. 35, no. 2, p. 12.
Murphy, E.C., 2011, Spikes on gamma ray logs southwestern North Dakota: North Dakota Geological Survey Geologic Investigation No. 138. GI-138
Murphy, E.C., 2011, Uranium concentrations in groundwater southwestern North Dakota: North Dakota Geological Survey Geologic Investigation No. 139. GI-139
Murphy, E.C., 2015, Uranium in North Dakota: North Dakota Geological Survey Geologic Investigation No. 184, 47 p. GI-184
Noble, E.A., 1973, Uranium in coal, in Mineral and water resources of North Dakota: North Dakota Geological Survey Bulletin 63 p. 80-85.
Volcanic Ash
Several volcanic ash, or pumicite, beds (tuffs) are known to be present in western and central North Dakota. These tuffs range in age from 70 to 20 million years and are believed to have originated from volcanoes in south-central and western Montana and northern Wyoming. Numerous additional volcanic ash beds are likely present in the Pierre and Niobrara formations but most, if not all, of the glass shards have been altered to bentonitic clay. These tuffs generally are thought to be the result of single or related airfall events that likely deposited a rather thin layer of volcanic dust across a wide area. In turn, this volcanic dust was transported by wind and rain into streams and redeposited in local basins, commonly lakes, as thick tuffs. As a result of this reworking, an inch or less of volcanic dust may result in 20 feet or more of tuff.
The glass grains or shards in tuffs have been used in the commercial manufacturing of abrasives; cleansers; scouring or polishing compounds; concrete admixtures; glazes for pottery, brick and tile; glass wool; enamels; lightweight products; fertilizer; asphalt constituents; acoustical tile; sweeping compounds; paint filler; insecticide carrier; a catalyst carrier in the chemical industry; absorptive packing material; and in purification of lard and tallow. Most of the ash mined in the United States is used in the manufacture of lightweight concrete.
Tuffs have been discovered in Emmons, Sioux, Stark, Dunn, Slope, and McKenzie counties. The most well-known tuff deposit is the Linton Ash which has been actively promoted for a period of twenty years but has undergone little development.
Linton Ash
T.W. Stanton was the first to identify volcanic ash in the Linton area in 1917. The Linton Ash occurs in the lower part of the Fox Hills Formation and consists of 25 feet of fine-grained, moderately indurated tuff. There are approximately 500 million tons of ash in a 4,000-acre area around Linton. A number of companies tested Linton ash to determine if it would fulfill their needs, including the Weyerhauser Corporation, General Electric, Corning Glass, and the Minnesota Mining and Manufacturing Company (3M). In 1993, 3M obtained a patent on an oil containment boom that contained volcanic ash. Linton ash had been used in the development of the prototype.
Breien Ash
Laird and Mitchell (1942) were first to note the presence of volcanic ash in northern Sioux County, east of the town of Breien. They reported a layer of ash was present in the basal portion of the Hell Creek Formation, the stratigraphic position of this ash was later confirmed by Frye (1967) and Murphy and others (1995). Frye noted the ash had a rhyolitic composition and that 98% of the deposit was silt-sized glass shards. As first noted by Feldmann (1972), the ash in the Breien area appears coarser-grained than ash near Linton. The Breien ash is 20 to 25 feet thick in a cut on the north side of the Cannonball River. This ash contains 72% silica and 13.5% aluminum oxide. The Breien ash deposit extends over an area of at least 160 acres and contains approximately 9 million tons of ash.
Cannon Ball Ash
In 1972, Feldmann identified an ash in the upper portion of the Fox Hills Formation near the town of Cannon Ball in northern Sioux County. Feldmann speculated that the Cannon Ball, Linton, and Breien ashes might be the result of one ash fall. He theorized that the ash gets progressively lower stratigraphically from west to east because it is reflecting the paleo-surface at the time of the ash fall event. In other words, when the ash was blown in from Montana or Wyoming (likely source areas) the edge or near-shore portions of the Interior Seaway (Hell Creek and Fox Hills Formations) were in the Breien area and the deeper, offshore portion of the seaway (Pierre Formation) was east of Linton. Feldmann supported this theory by noting the Breien, Cannon Ball, and Linton ashes are lithologically similar and are progressively finer-grained from west to east.
Marmarth Ash
The Marmarth Ash is present in the uppermost part of the Hell Creek Formation northeast of the town of Marmarth in Slope County. The Marmarth Ash consists of approximately 6 feet of unaltered (white) tuff which is bounded by bentonite. The bentonite was formed by the alteration of the volcanic glass. This tuff could only be traced through portions of two sections indicating it may only extend over an area of 600 acres.
Sentinel Butte tuff
The Sentinel Butte tuff or blue bed occurs near the middle of the Sentinel Butte Formation in McKenzie County. The Sentinel Butte tuff is best exposed in the North Unit of the Theodore Roosevelt National Park and extends to the northern edge of McKenzie County, an area of at least 600 square miles. The Sentinel Butte tuff is up to 23 feet thick commonly comprised of an unaltered tuff layer ranging in thickness from 0 to 6 feet which is bounded by bentonite layers that range up to 12 feet thick.
Killdeer Mountains
The Arikaree caprock of the Killdeer Mountains consists of tuffaceous sandstone and siltstone that is interbedded with thin layers of freshwater carbonates. Dating techniques performed on a tuffaceous layer 200 feet below the top of South Killdeer Mountain indicated that volcanic ash at that horizon was deposited approximately 25 million years ago. The presence of interbedded carbonates in the tuff would make mining and processing of this material very difficult. The tuffaceous rocks are present throughout the 4,800-acre area of the Killdeer Mountains.
Antelope Creek Ash
A 2-to 3-foot tuff layer (Antelope Creek Ash) is present in the lower portion of the Brule Formation in the Little Badlands southwest of Dickinson. This tuff is very fine-grained and initial attempts at dating this layer failed. The Antelope Creek Ash may extend over an area of 2000 acres.
Selected References for North Dakota Volcanic Ash
Bluemle, J.P., 1984, Geology of Emmons County, North Dakota: North Dakota Geological Survey Bulletin 66, Part 1, 69 p. B-66
Feldmann, R.M., 1972, Stratigraphy and paleocology of the Fox Hills Formation (Upper Cretaceous) of North Dakota: North Dakota Geological Survey Bulletin 61, 65 p. B-61
Forsman, N.F., 1985, Petrology of the Sentinel Butte Formation (Paleocene), North Dakota: Unpublished Doctoral Thesis, University of North Dakota, 222 p.
Forsman, N.F., 1984, Durability and alteration of some Cretaceous and Paleocene pyroclastic glasses in North Dakota: Journal of Noncrystalline solids, Vol. 67, p. 449-461.
Forsman, N.F., 1992, Tuffs in North Dakota, in Proceedings of the F.D. Holland, Jr., Geological Symposium, Erickson, J.M. and Hoganson, J.W., eds: North Dakota Geological Survey Miscellaneous Series No. 76, p. 267-273. MS-76
Frye, C.I., 1967, The Hell Creek Formation in North Dakota: PhD Thesis, University of North Dakota, Grand Forks, 211 p.
Laird, W.M. and Mitchell, R.H., 1942, The geology of the southern part of Morton County, North Dakota: North Dakota Geological Survey Bulletin 14, 42 p. B-14
Larsen, R.A., 1988, Major and trace element characterization and correlation of the Sentinel Butte ash/bentonite (Paleocene): M.S. Thesis, University of North Dakota, 162 p.
Manz, O.E., 1962, Investigation of pozzolanic properties of the Cretaceous volcanic ash deposit near Linton, North Dakota: North Dakota Geological Survey Report of Investigation No. 38, 42 p. RI-38
Murphy, E.C., 2005, Volcanic Ash in South-Central North Dakota: Department of Mineral Resources Geo News Newsletter, vol. 32, no. 2, p. 1-4.
Murphy, E.C., Hoganson, J.W., and Forsman, N.F., 1993, The Chadron, Brule, and Arikaree Formations in North Dakota: North Dakota Geological Survey Report of Investigation No. 96, 144 p. RI-96